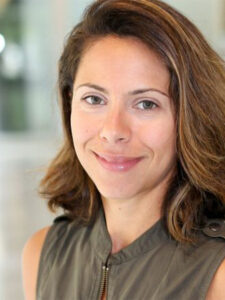
Marianne Hatzopoulou
Professor
Department Chair
Department of Civil & Mineral Engineering
Tier 1 Canada Research Chair in Transportation Decarbonization and Air Quality
Director, Positive Zero Transport Futures
Head of the Transportation and Air Quality research group TRAQ
Email: marianne.hatzopoulou@utoronto.ca
Office: GB107
Tel: 416-978-0864
Expertise:
Areas of Specialization:
Environmental Analysis: Traffic-related Air Pollution / Urban Air Quality
Biography
Marianne Hatzopoulou is Professor in the Department of Civil and Mineral Engineering at the University of Toronto and Tier 1 Canada Research Chair in Transport Decarbonization and Air Quality. She leads the Transportation and Air Quality (TRAQ) research group studying the interactions between transportation, air quality, climate change, and public health; she published 150 publications on these topics. Prof. Hatzopoulou is also the Director of Positive Zero Transport Futures, a living lab ecosystem for testing transport decarbonization innovations with positive societal outcomes. Prof. Hatzopoulou is on the Canadian team of researchers who were the 2021 recipients of the NSERC Brockhouse Canada Prize for Interdisciplinary Research in Science and Engineering. In 2022, she received the University of Toronto Engineering Alumni Network 2T5 Mid-Career Achievement Award. She is an associate editor of the journal Transportation Research Part D: Transport and Environment and the current chair of the Transportation Research Board Standing Committee on “Air Quality and Greenhouse Gas Mitigation” (2023-2026).
Background
Her research bridges transportation and environmental analysis; and her main expertise is in modelling of road transport emissions and urban air quality as well as evaluating population exposure to air pollution. She is interested in capturing the interactions between the daily activities and travel patterns of urban dwellers and the generation and dispersion of traffic emissions in urban environments. She leads an active research group focusing on modelling traffic emissions and near-road air quality as well as near-road air pollution monitoring and characterization.
The research of the TRAQ research group was recently featured in the documentary Something in the Air, The Nature of Things: https://www.cbc.ca/natureofthings/episodes/something-in-the-air
Research Interests
- Traffic-Related Air Pollution
- Urban Air Quality
- Transportation Engineering
- Transportation Planning
Education
BSc (Physics, American University of Beirut, 1999)
MSc (Envtal Technology, Civil Eng., American University of Beirut, 2001)
PhD (Transportation Engineering, Civil Eng., University of Toronto, 2008)
Postdoctoral training (University of Toronto, 2009)
Postdoctoral training (Massachusetts Institute of Technology, 2010)
- Canada Research Chair in Transport Decarbonization and Air Quality
- Associate Chair-Graduate Studies, Department of Civil & Mineral Engineering
- Director - Positive Zero Transport Futures
- Head - Air Quality Group, U of T Data Sciences Institute: Research and Academics Committee
- Collaborating Researcher - U of T Climate Positive Initiative
- Researcher - Centre for Automated and Transformative Transportation Systems (CATTS)
- Researcher - City Logistics for the Urban Economy (CLUE)
- Affiliate - Centre for Climate Science and Engineering (CSE)
- Affiliate - U of T Mobility Network
- Affiliate - U of T School of Cities
- Affiliate - GTHA Smart Freight Centre
- Head - Transportation and Air Quality Research Group (TRAQ)
- Contributor: The OECD Forum Network

Research in Images

UrbanScanner platform for measuring air quality and capturing images of the urban environment developed by TRAQ group in collaboration with industrial partner


PEMS (Portable Emissions Monitoring System) testing of exhaust for a gasoline direct injection SUV while driving in Toronto


Distribution of ambient NO2 across the Montreal Island


Distribution of Black Carbon levels across Toronto


Distribution of ultrafine particles in Toronto


Black Carbon across cycling routes in Toronto


Ultrafine particles along cycling routes in Toronto


Testing Aeroqual S500 sensors againts fixed station (RSQA) in Montreal

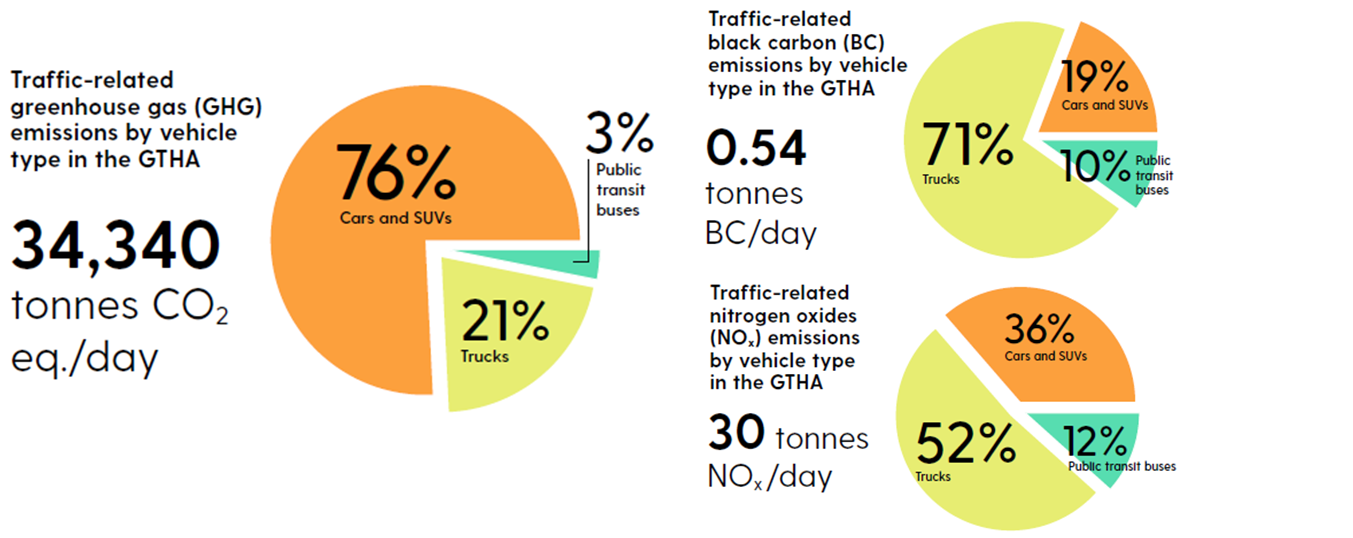
Traffic-related emission inventory in the Greater Toronto and Hamilton Area
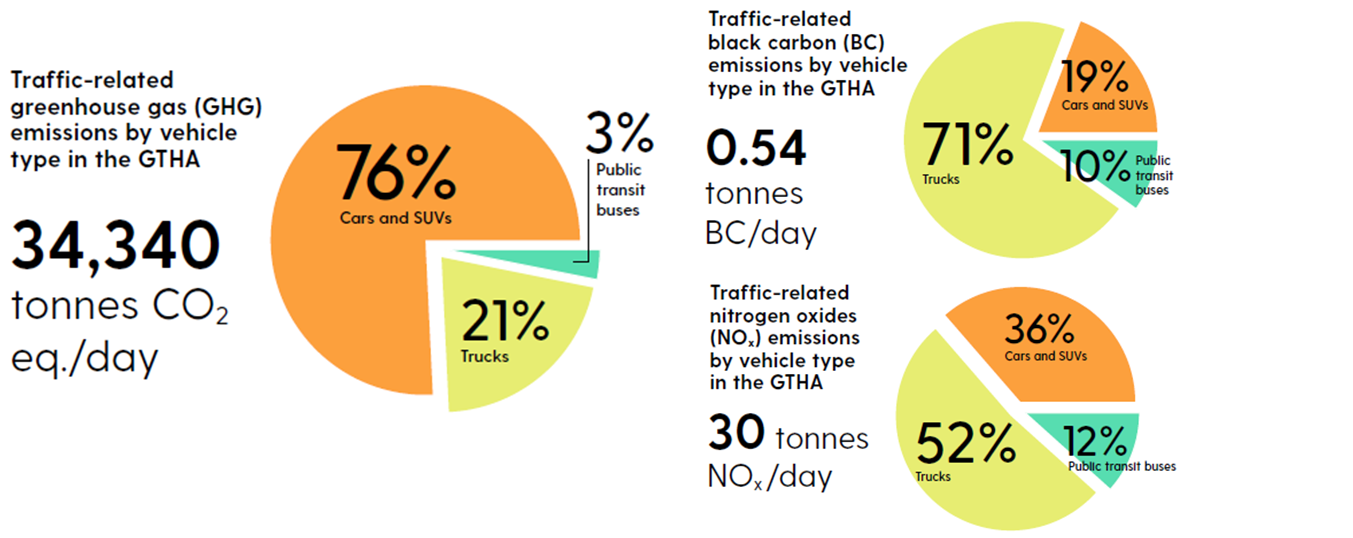
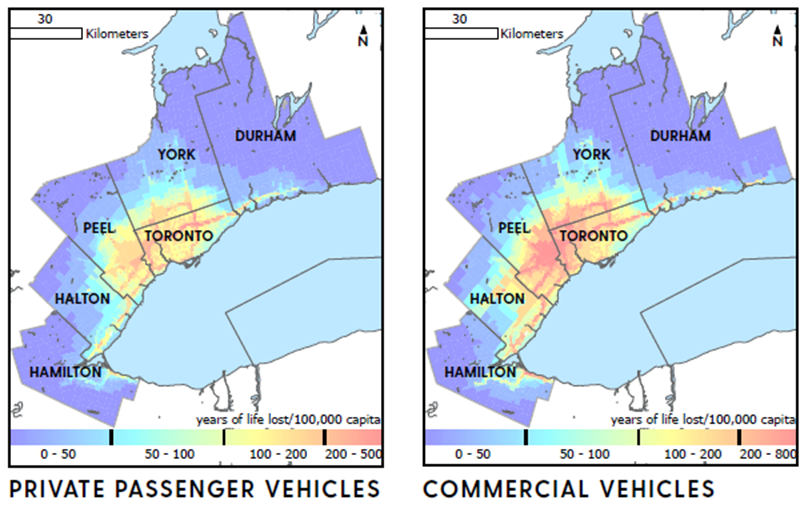
Annual number of years of life lost per 100,000 persons due to air pollution from passenger vehicles (332 premature deaths per year) and commercial vehicles (407 premature deaths per year)
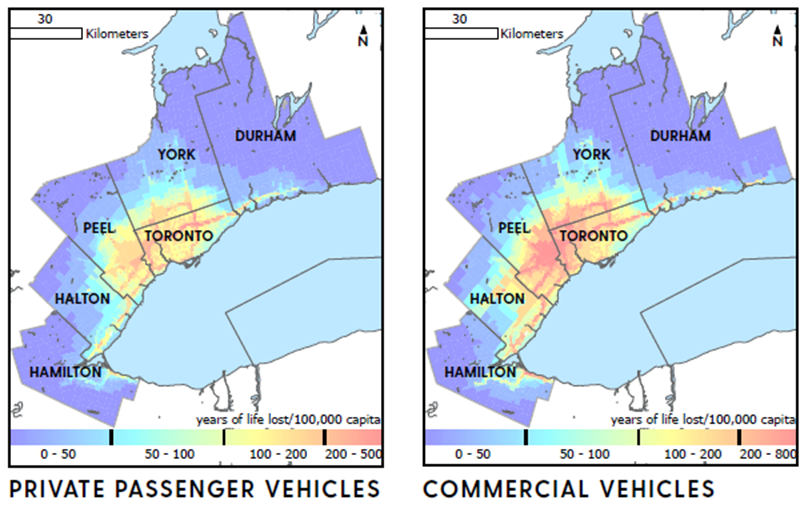
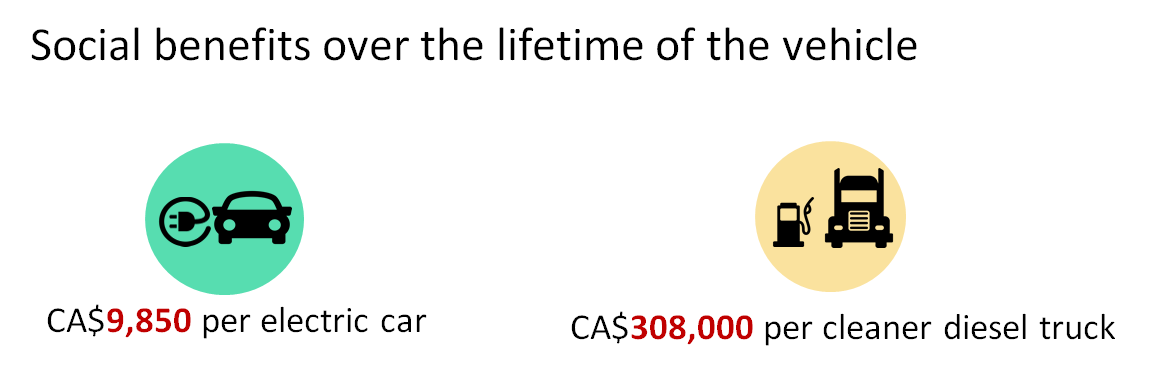
Monetized social benefits
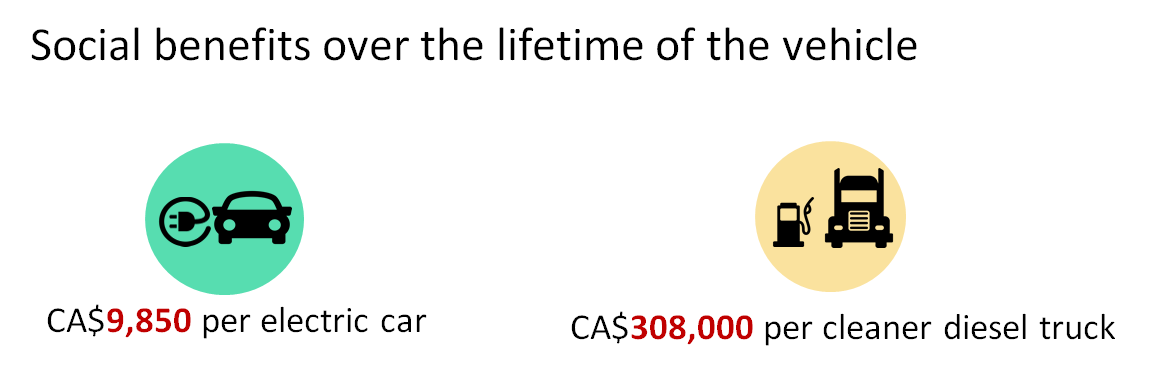
Please find descriptions of research studies on the Positive Zero website:
Publications
2021
- Minet, L., A. Wang, M. Hatzopoulou. 2021. Health and climate incentives for the deployment of cleaner on-road vehicle technologies. Environmental Science and Technology, 55, 10, 6602–6612.
- Xu, J., R. Tu, U. Ahmed, G. Amirjamshidi, M. Hatzopoulou, M. Roorda. 2021. An Eco-Score System Incorporating Driving Behavior, Vehicle Characteristics, and Traffic Conditions, Transportation Research Part D, 95: 102866
- Stieb, D., G.J. Evans, T.M. To, P.S.J. Lakey, M. Shiraiwa, M, Hatzopoulou, L. Minet, J.R. Brook, R.T. Burnett, S.A. Weichenthal. 2021. Within-city Variation in Reactive Oxygen Species from Fine Particle Air Pollution and COVID-19. American Journal of Respiratory and Critical Care Medicine, Accepted.
- Fallah-Shorshani, M., M. Franklin, M. Hatzopoulou. 2021. Evaluation of aggregate and individual vehicle activity on emissions models and their impact on air quality. Atmospheric Pollution Research, 12 (5): 101052.
- Wang, A., J. Xu, M. Zhang, M. Adams, M. Hatzopoulou. 2021. Near-Road Air Quality Modelling that Incorporates Input Variability and Model Uncertainty. Environmental Pollution, 284: 117145.
- Liu, L., T. Oiamo. D. Rainham, H. Chen, M. Hatzopoulou, J.R. Brook, H. Davies, S. Goudreau, A. Smargiassi. 2021. Integrating random forests and propagation models for high-resolution noise mapping. Environmental Research, 195:110905.
- Yankoty, L., P. Gamache, C. Plante, S. Goudreau, C. Blais, S. Perron, M. Fournier, M. Ragettli, M. Fallah-Shorshani, M. Hatzopoulou, Y. Liu, A. Smargiassi. 2021. Long-term residential exposure to environmental/transportation noise and the incidence of myocardial infarction. International Journal of Hygiene and Environmental Health, 232(1):113666
2020
- Zhang, Z., S. Weichenthal, J. Kwong, R.T. Burnett, M. Hatzopoulou, M. Jerret, A. Van Dokelaar, L. Bai, R. Martin, R. Copes, H.Lu, P. Lakey, M. Shiraiwa, H. Chen. 2020. Long-term exposure to iron and copper in fine particulate air pollution and their combined impact on reactive oxygen species concentration in lung fluid: a population-based cohort study of cardiovascular disease incidence and mortality in Toronto, Canada. International Journal of Epidemiology, 10.1093/ije/dyaa230.
- Zalzal, J., I. Alameddine, M. El-Fadel, S. Weichenthal, M. Hatzopoulou. 2020. Drivers of seasonal and annual air pollution exposure in a complex urban environment with multiple source contributions. Environmental Monitoring and Assessment,192, 1-23.
- Karumanchi, S., J. Siemiatycki, L. Richardson, M. Hatzopoulou, E. Lequy. 2021. Spatial and temporal variability of airborne ultrafine particles in the Greater Montreal area: Results of monitoring campaigns in two seasons. Science of The Total Environment, 771:144652.
- Tu, R., J. Xu, A. Wang, Z. Zhai, M. Hatzopoulou. 2020. Effects of ambient temperature and cold starts on excess NOx emissions in a gasoline direct injection vehicle. Science of The Total Environment, 760:143402
- Wang, A., J. Xu, R. Tu, M. Saleh, M. Hatzopoulou. 2020. Potential of machine learning for prediction of traffic related air pollution. Transportation Research Part D, 88:102599.
- Biel, C. Danieli, M. Shekarrizfard, L. Minet, M. Abrahamowicz, J. Baumgartner, R. Liu, M. Hatzopoulou, S. Weichenthal. 2020. Acute cardiovascular health effects in a panel study of personal exposure to traffic-related air pollutants and noise in Toronto, Canada. Scientific Reports, 10(1).
- El-Faseeh, L., R. Tu, B. Farooq, M. Hatzopoulou. 2020. Greenhouse Gas Emission Prediction on Road Network Using Deep Sequence Learning. Transportation Research Part D, 88: 102593.
- Buteau, S., S. Belkaibech, M. Bilodeau-Bertrand, M. Hatzopoulou, A. Smargiassi, N. Auger. 2020. Association between Kawasaki disease and prenatal exposure to ambient and industrial air pollution: A population-based cohort study. Environmental Health Perspectives, 128 (10): 107006.
- Saleh, M. and M. Hatzopoulou, 2020. Greenhouse gas emissions attributed to empty kilometers in automated vehicles. Transportation Research Part D, 88: 102567
- Xu, J., R. Tu, A. Wang, Z. Zhai, M. Hatzopoulou. 2020. Generation of spikes in ultrafine particle emissions from a gasoline direct injection vehicle during on-road emission tests. Environmental Pollution, 276, 115695.
- Lavigne, E., I. Lima, M. Hatzopoulou, K. Van Ryswyk, A. van Donkelaar, R.V. Martin, H. Chen, D.M. Stieb, R.T. Burnett, S. Weichenthal. 2020. Ambient Ultrafine Particle Concentrations and Incidence of Childhood Cancers. Environment International, 145, 106135.
- Ganji, A., L. Minet, S. Weichenthal, M. Hatzopoulou. 2020. Predicting Traffic-Related Air Pollution Using Feature Extraction from Built Environment Images. Environmental Science and Technology, 54, 17, 10688–10699.
- Djavadian, S., R. Tu, B. Farooq, M. Hatzopoulou. 2020. Multi-Objective Eco-Routing for Dynamic Control of Connected and Automated Vehicles. Transportation Research Part D, 87: 102513
- Zhao N, Smargiassi A, Hatzopoulou M, Colmegna I, Hudson M, Fritzler MJ, Awadalla P, Bernatsky S. 2020. Long-term exposure to a mixture of industrial SO2, NO2, and PM and anti-citrullinated protein antibody positivity. Environmental Health, 19 (1): 86. doi: 10.1186/s12940-020-00637-3.
- Zhai, Z., R. Tu, J. Xu, A. Wang, M. Hatzopoulou. 2020. Capturing the Variability in Instantaneous Vehicle Emissions Based on Field Test Data. Atmosphere, 11 (7): 765.
- Morency, P. C. Plante, A.S. Dube, S. Goudreau, C. Morency, P.L. Bourbonnais, N. Eluru, L.F. Tetreault, M. Hatzopoulou, N.C. Iraganaboina, T. Bhowmik, A. Smargiassi. 2020. The Potential Impacts of Urban and Transit Planning Scenarios for 2031 on Car Use and Active Transportation in a Metropolitan Area. International Journal of Environmental Research and Public Health, 17 (14), 5061.
- Tu, R., Y. Gai, D. Posen, B. Farooq, M. Hatzopoulou. 2020. Electric Vehicle Charging Optimization to Minimize Marginal Greenhouse Gas Emissions from Power Generation, Applied Energy, 277: 115517.
- Gai. Y., L. Minet, I.D. Posen, M. Hatzopoulou. 2020. Health and Climate Benefits of Electric Vehicle Deployment in the Greater Toronto and Hamilton Area, Environmental Pollution, 265 (Part A): 114983.
- Xu, J., A. Wang, N. Schmidt, M. Adams, M. Hatzopoulou. 2020. A Gradient Boost Approach for Predicting Near-Road Ultrafine Particle Concentrations Using Detailed Traffic Characterization, Environmental Pollution, 265 (Part B): 114777.
- Plante, C., P. Morency, M. Hatzopoulou, C. Morency, N. Eluru, LF Tetreault, S. Goudreau, PL Bourbonnais, T. Bhowmik, M. Shekarrizfard, N C Iraganaboina, W. Requia, A. Smargiassi. 2020. Environmental and health impacts of transportation and land use scenarios in 2061. Environmental Research, 187: 109622.
- Wang, A. R. Tu, Y. Gai, L. G. Pereira, I. D. Posen, E. J. Miller, M. Hatzopoulou. 2020. Capturing uncertainty in emission estimates related to vehicle electrification and implications for metropolitan greenhouse gas emission inventories, Applied Energy, Volume 265, May 2020, 114798.
- Shekarrizfard, M., L. Minet, M. Fallah-Shorshani, E. Miller, B. Yusuf, S. Weichenthal, M. Hatzopoulou. 2020. Influence of travel behaviour and daily mobility on exposure to traffic-related air pollution, Environmental Research, Volume 184, May 2020, 109326.
- Minet, L., T. Chowdbury, A. Wang, Y. Gai, I.D. Posen, M. Roorda, M. Hatzopoulou. 2020. Quantifying the air quality and health benefits of greening freight movements, Environmental Research, Volume 183, April 2020, 109193.
- Xu, J., M. Saleh, M. Hatzopoulou. 2020. A machine learning approach capturing the effects of driving behaviour and driver characteristics on trip-level emissions, Atmospheric Environment, Volume 224, March 2020, 117311.
- Shi, T., Dirienzo, N., Requia, W. J., Hatzopoulou, M., Adams, M. D. 2020. Neighbourhood scale nitrogen dioxide land use regression modelling with regression kriging in an urban transportation corridor. Atmospheric Environment, Volume 223, February 2020, 117218.
- Weichenthal, S., T. Olaniyan, T. Christidis, E. Lavigne, M. Hatzopoulou, K. Van Ryswyk, M. Tjepkema, R. Burnett. 2019. Within-city spatial variations in ambient ultrafine particle concentrations and incident brain tumors in adults. Epidemiology, 31(2): 177–183.
- Liu Y, S. Goudreau,T. Oiamo, D. Rainham, M. Hatzopoulou, H. Chen, H. Davies, M. Tremblay, J. Johnson, A. Bockstael. 2019. Comparison of land use regression and random forests models on estimating noise levels in five Canadian cities. Environmental Pollution, Volume 256, January 2020, 113367.
2019
- Stogios, C., D. Kasraian, M. Roorda, M. Hatzopoulou. 2019. Simulating impacts of automated driving behaviour and traffic conditions on vehicle emissions, Transportation Research Part D, 76: 176-192.
- Ganji, A., M. Shekarrizfard, A. Harpalani, J. Coleman, M. Hatzopoulou. 2019. Methodology for spatio-temporal predictions of traffic counts across an urban road network and generation of an on-road greenhouse gas emission inventory, Computer-Aided Civil and Infrastructure Engineering, https://doi.org/10.1111/mice.12508.
- Tu R., A. Wang, M. Hatzopoulou. 2019. Improving the Spatial Accuracy of Regional Emission Inventories and Investigating the Transferability of Emission Modeling Approaches across Different Cities, Journal of the Air and Waste Management Association, 69 (11): 1377-1390.
- Lavigne, E., I. Lima, M. Hatzopoulou, K. Van Ryswyk, ML. Decou, W. Luo, A. van Donkelaar, R.V. Martin, H. Chen, D. M. Stieb, E. Crighton, A. Gasparrini, A. S. Yasseen III, R. T. Burnett, M. Walker, S. Weichenthal. 2019. Spatial variations in ambient ultrafine particle concentrations and risk of congenital heart defects, Environment International, Accepted.
- Hong, K.Y., P.O. Pinheiro, L. Minet, M. Hatzopoulou, S. Weichenthal. 2019. Extending the spatial scale of land use regression models for ambient ultrafine particles using satellite images and deep convolutional neural networks. Environmental Research, Accepted.
- Gai, Y., A. Wang, L. Pereira, M. Hatzopoulou, I. D. Posen. 2019. Marginal greenhouse gas emissions of Ontario’s electricity system and the implications of electric vehicle charging. Environmental Science and Technology, Accepted.
- Xu J., M. Saleh, A. Wang, R. Tu, M. Hatzopoulou. 2019. Towards a Canadian Version of the MOVES Model: Capturing Driving Behaviours in Greater Toronto and Comparison against US Defaults. Transportation Research Part D, Accepted.
- Tu R., L. Alfaseeh., S. Djavadian., M. Saleh., B. Farooq., M. Hatzopoulou. 2019. Quantifying the impacts of dynamic control in connected and automated vehicles on greenhouse gas emissions and urban NO2 concentrations Transportation Research Part D, Accepted.
- Zalzal J., I. Alameddine, C. El Khoury, L. Minet, M. Shekarrizfard, S. Weichenthal, M. Hatzopoulou. 2019. Assessing the transferability of land use regression models for ultrafine particles across two Canadian cities. Science of the Total Environment, 662:722-734.
- Weichenthal, S., M. Shekarrizfard, A. Traub, R. Kulka, K. Al-Rijleh, S. Anowar, G. Evans, M. Hatzopoulou. 2019. Within-city spatial variations in multiple measures of PM2.5 oxidative potential in Toronto, Canada. Environmental Science and Technology, 53(5): 2799-2810.
- Waraich, A., S. Anowar, T. Tenaglia, T. Sider, A. Alam, N. Shabaanzaadeh, M. Hatzopoulou, N. Eluru. 2019. Disaggregate-level simulation of bus transit emissions in a large urban region. International Journal of Sustainable Transportation, https://doi.org/10.1080/15568318.2019.1579009
- Lavigne, E., J. Donnelle, M. Hatzopoulou, K. Van Ryswyk, A. van Donkelaar, et al. 2019. Spatiotemporal variations in ambient ultrafine particles and the incidence of childhood asthma. American Journal of Respiratory and Critical Care Medicine, https://doi.org/10.1164/rccm.201810-1976OC.
- Chamseddine, A., I. Alameddine, M. Hatzopoulou, M. El Fadel. 2019. Seasonal variation of air quality in hospitals with indoor–outdoor correlations. Building and Environment, 148: 689-700.
- Weichenthal, S., M. Hatzopoulou, M. Brauer. 2019. A picture tells a thousand…exposures: Opportunities and challenges of deep learning image analyses in exposure science and environmental epidemiology. Environment International, 122: 3-10.
2018
- Minet, L., J. Stokes, J. Scott, M.F. Valois, J. Xu, S. Weichenthal, M. Hatzopoulou. 2018. Should traffic-related air pollution be considered when designing urban bicycle networks? Accepted, Transportation Research Part D, 65: 736-749.
- Anowar, S., B. Dey, N. Eluru, M. Hatzopoulou. 2018. Accommodating exogenous variable and decision rule heterogeneity in discrete choice models: Application to bicyclist route choice. PLOS ONE, https://doi.org/10.1371/journal.pone.0208309.
- Shekarrizfard, M., M.F. Valois, S. Weichenthal, M.S Goldberg, M. Fallah-Shorshani, L. Deville-Cavellin, D. Crouse, M.E. Parent, M. Hatzopoulou. 2018. Investigating the effects of multiple exposure measures to traffic-related air pollution on the risk of breast and prostate cancer. Journal of Transport & Health, 11: 34-46.
- Al-Rijleh, K., A. Alam, R. Foti, P. Gurian, S. Spatari, M. Hatzopoulou. 2018. Strategies to achieve deep reductions in metropolitan transportation GHG emissions: The case of Philadelphia. Transportation Planning and Technology, 41 (8): 797-815.
- Bai, L, S. Weichenthal, J.C. Kwong, R. Burnett, M. Hatzopoulou, M. Jerrett, A. van Donkelaar, R. Martin, K. Van Ryswyk, H. Lu, A. Kopp, H. Chen. 2018. Associations of long-term exposure to ultrafine particles and nitrogen dioxide with increased incidence of congestive heart failure and acute myocardial infarction. American Journal of Epidemiology, 188 (1): 151-159.
- Fallah-Shorshani, M., M. Hatzopoulou, N.A. Ross, Z. Patterson, S. Weichenthal. 2018. Evaluating the impact of neighborhood characteristics on differences between residential and mobility-based exposures to outdoor air pollution. Environmental Science and Technology, 52 (18):10777-10786.
- Fallah-Shorshani, M., L. Minet, R. Liu, C. Plante, S. Goudreau, T. Oiamo, A. Smargiassi, S. Weichenthal, M. Hatzopoulou. 2018. Capturing the spatial variability of noise levels based on a short-term monitoring campaign and comparing noise surfaces against personal exposures collected through a panel study. Environmental Research, 167: 662-672.
- Xu, J., J. Wang, N. Hilker, M. Fallah-Shorshani, M. Saleh, R. Tu, A. Wang, L. Minet, C. Stogios, G. Evans, M. Hatzopoulou. 2018. Comparing emission rates derived from a model with a plume-based approach and quantifying the contribution of vehicle classes to on-road emissions and air quality. Journal of the Air & Waste Management Association, 5:1-16.
- Weichenthal, S., M. Shekarrizfard, R. Kulka, P.S.J. Lakey, K. Al-Rijleh, S. Anowar, M. Shiraiwa, M. Hatzopoulou. 2018. Spatial variations in the estimated production of reactive oxygen species in the epithelial lung lining fluid by iron and copper in fine particulate air pollution. Environmental Epidemiology, 2 (3): e20 (p1-9).
- Wang, A., C. Stogios, Y. Gai, J. Vaughan, G. Ozonder, S. Lee, D.I, Posen, E.J. Miller. M. Hatzopoulou. 2018. Automated, electric, or both? Investigating the effects of transportation and technology scenarios on metropolitan greenhouse gas emissions. Sustainable Cities and Society, 50: 524-533.
- Tu, R., I. Kamel, A. Wang, B. Abdulhai, M. Hatzopoulou. 2018. Development of a hybrid modeling approach for the generation of an urban on-road transportation emission inventory. Transportation Research Part D, 62: 604-618.
- Minet, L., R. Liu, M.F. Valois, J. Xu, S. Weichenthal, M. Hatzopoulou. 2018. A comparison of air pollution exposure surfaces developed via on-road mobile monitoring and short-term stationary sidewalk measurements. Environmental Science and Technology, 52 (6): 3512-3519.
- Buteau, S., M.S Goldberg, R. Burnett, A. Gasparrini, M.F Valois, J.M Brophy, D.L Crouse, M. Hatzopoulou. 2018. Associations between ambient air pollution and daily mortality in a cohort of congestive heart failure: Case-crossover and nested case-control analyses using a distributed lag nonlinear model. Environment International, 113: 313-324.
- Xu, J., M. Turchet, N. Hilker, M. Fallah-Shorshani, G. Evans, M. Hatzopoulou. 2018. Contrasting direct use of data from traffic radar and video cameras with traffic simulation software in estimation of vehicle emissions. Transportation Research Part D, 62: 90-101.
- Tu, R., I. Kamel, B. Abdulhai, M. Hatzopoulou. 2018. Reducing transportation greenhouse gas emissions through the development of policies targeting high-emitting trips. Transportation Research Record, Journal of the Transportation Research Board. Accepted, https://doi.org/10.1177/0361198118755714.
- Bai L, H. Chen, M. Hatzopoulou, M. Jerrett, JC. Kwong, RT. Burnett, A. van Donkelaar, R. Copes, RV. Martin, K. van Ryswyk, H. Lu, A. Kopp, S. Weichenthal. 2018. Exposure to ambient ultrafine particles and nitrogen dioxide and incident hypertension and diabetes. Epidemiology, 29 (3): 323-332.
- Louis-François, T., N. Eluru, M. Hatzopoulou, P. Morency, C. Plante, C. Morency, F. Reynaud, M. Shekarrizfard, Y. Shamsunnahar, A. Faghih Imani, L. Drouin, A. Pelletier, S. Goudreau, F. Tessier, L. Gauvin, A. Smargiassi. 2018. Estimating the health benefits of planned public transit investments in Montreal. Environmental Research, 160: 412-419.
2017
- Anowar, S., M. Hatzopoulou, N. Eluru. 2017. Quantifying the value of a clean ride: How far would you bicycle to avoid exposure to traffic-related air pollution?. Transportation Research Part A, 105: 66-78.
- Minet, L., R. Gehr, M. Hatzopoulou. 2017. Capturing the sensitivity of land-use regression models to short-term mobile monitoring campaigns using air pollution micro-sensors. Environmental Pollution, 230: 280-290.
- Goldberg, M.S., F. Labreche, S. Weichenthal, E. Lavigne, M.F. Valois, M. Hatzopoulou, K. Van Ryswyck, M. Shekarrizfard, P.J. Villeneuve, D. Crouse, M.E., Parent. 2017. The association between the incidence of postmenopausal breast cancer and concentrations at street-level of nitrogen dioxide and ultrafine particles. Environmental Research, 5 (158): 7-15.
- Fallah-Shorshani, M., M. Hatzopoulou, M. Shekarrizfard. 2017. Evaluation of regional and local atmospheric dispersion models for the analysis of traffic-related air pollution in urban areas. Atmospheric Environment, 167: 270-282.
- Alam, A., M. 2017. Hatzopoulou. Modeling transit bus emissions using MOVES: Validation of default distributions and embedded drive cycles with local data. ASCE Journal of Transportation Engineering, Part A: Systems, 143 (10): 04017049 1-9.
- Harik G., M. El-Fadel, A. Shihadeh, I. Alameddine, M. Hatzopoulou. 2017. Is in-cabin exposure to carbon monoxide and fine particulate matter amplified by the vehicle’s self-pollution potential? Quantifying the rate of exhaust intrusion. Transportation Research Part D, 54: 225-238.
- Weichenthal, S., L. Bai, M. Hatzopoulou, K. Van Ryswyk, J.C. Kwong, M. Jerrett, A. van Donkelaar, R.V. Martin, R.T. Burnett, H. Lu, H. Chen. 2017. Long-term exposure to ambient ultrafine particles and respiratory disease incidence in Toronto, Canada: A cohort study. Environmental Health, 16:64.
- Weichenthal, S., E, Lavigne, M.F Valois, M. Hatzopoulou, K. Van Ryswyk, M. Shekarrizfard, P. Villeneuve, M. Goldberg, M.E Parent. 2017. Spatial variations in ambient ultrafine particle concentrations and the risk of incident prostate cancer: A case-control study. Environmental Research, 156: 374-380.
- Buteau, S. M. Hatzopoulou, D. Crouse, A. Smargiassi, R. Burnett, T. Logan, L. Deville Cavellin, M. Goldberg. 2017. Comparison of spatiotemporal prediction models of daily exposure of individuals to ambient nitrogen dioxide and ozone in Montreal, Canada. Environmental Research, 156: 201-230
- Hatzopoulou, M., M.F Valois, I. Levy, C. Mihele, G. Lu, S. Bagg, L. Minet, J. Brook. 2017. Robustness of land-use regression models developed from mobile air pollutant measurements. Environmental Science and Technology, 51 (7): 3938-3947.
- Shekarrizfard, M., A. Faghih-Imani, LF. Tetreault, S. Yasmin, P. Morency, C. Plante, L. Drouin, A. Smargiassi, N. Eluru, M. Hatzopoulou. 2017. Modelling the spatio-temporal distribution of ambient nitrogen dioxide and investigating the effects of public transit policies on population exposure. Environmental Modelling and Software, 91: 186-198.
- Shekarrizfard, M., A. Faghih-Imani, LF. Tetreault, S. Yasmin, F. Reynaud, P. Morency, C. Plante, L. Drouin, A. Smargiassi, N. Eluru, M. Hatzopoulou. 2017. Regional assessment of exposure to traffic-related air pollution: Impacts of individual mobility and transit investment scenarios. Sustainable Cities and Society, 29: 68-76.
- Fallah-Shorshani, M., M. Shekarrizfard, M. Hatzopoulou. 2017. Integrating a street-canyon model with a regional Gaussian dispersion model for improved characterisation of near-road air pollution. Atmospheric Environment, 153: 21-31.
- Addo, A. and M. Hatzopoulou. 2017. Harnessing the potential of AVL and APC data to simulate greenhouse gas emissions of an interregional bus route in Toronto, Canada. Transportation Research Record, Journal of the Transportation Research Board, 2627: 36-45.
2016
- Reynaud, F., T. Sider, M. Hatzopoulou, N. Eluru. 2016. Extending the Network Robustness Index to include emissions: a holistic framework for link criticality analysis for Montreal transportation system. Transportation Letters.
- Wang, A., J. Xu, M. Fallah-Shorshani, M. Hatzopoulou. 2016. Characterizing near-road air pollution using local-scale emission and dispersion models and validation against in-situ measurements. Atmospheric Environment, 142: 452-464.
- Xu, J., A. Wang, M. Hatzopoulou. Investigating near-road particle number concentrations along a busy urban corridor with varying built environment characteristics. 2016. Atmospheric Environment, 142: 171-180.
- Lawlor, S., M. Rabbat, T. Sider, N. Eluru, M. Hatzopoulou. 2016. Detecting convoys using license plate recognition data. IEEE Transactions on Signal and Information Processing over Networks, 2 (3): 391-405.
- Shekarrizfard, M., A. Faghih-Imani, D. Crouse, M. Goldberg, N. Ross, N. Eluru, M. Hatzopoulou. 2016. Individual exposure to traffic related air pollution across land-use clusters. Transportation Research Part D, 46: 339-350.
- Alam, A., M. Hatzopoulou. 2016. Deriving local operating distributions to estimate transit bus emissions across an urban network. Transportation Research Record: Journal of the Transportation Research Board, 2570: 57-68.
- Alamdeddine, I., L. Abi Esber, E. Abou Zeid, M. Hatzopoulou, M. El-Fadel. 2016. Operational and environmental determinants of in-vehicle CO and PM2.5 exposure. Science of the Total Environment, 551-552: 42-50.
- Weichenthal, S., K. Van Ryswyk, A. Goldstein, S. Bagg, M. Shekarrizfard, M. Hatzopoulou. 2016. A land use regression model for ambient ultrafine particles in Montreal, Canada: A comparison of linear regression and a machine learning approach. Environmental Research, 146: 65-72.
- Widener, M. and M. Hatzopoulou. 2016. Contextualizing research on transportation and health: A systems perspective. Journal of Transport and Health, 3 (3): 232-239.
- Ragettli, M., S. Goudreau, C. Plante, M. Fournier, M. Hatzopoulou, S. Perron, A. Smargiassi. 2016. Statistical modeling of the spatial variability of environmental noise levels in Montreal, Canada, using noise measurements and land use characteristics. 2016. Journal of exposure science and environmental epidemiology, 26 (6): 597-605.
- Deville Cavellin, L., S. Weichenthal, R. Tack, M. Ragettli, A. Smargiassi, M. Hatzopoulou. 2016. Investigating the use of portable air pollution sensors to capture the spatial variability of traffic related air pollution. Environmental Science & Technology, 50 (1): 313-320.
- Shekarrizfard, M., A. Faghih-Imani, M. Hatzopoulou. 2016. An examination of population exposure to traffic related air pollution: Comparing spatially and temporally resolved estimates against long-term average exposures at the home location. Environmental Research, 147: 435-444.
- Farrell, W., S. Weichenthal, M.F. Valois, M. Goldberg, M. Shekarrizfard, M. Hatzopoulou. 2016. Near-roadway air pollution across a spatially extensive road and cycling network. Environmental Pollution, 212: 498-507.
- Weichenthal, S., K. Van Ryswyk, A. Goldstein, M. Shekarrizfard, M. Hatzopoulou. 2016. Characterizing the spatial distribution of ultrafine particles in Toronto, Canada: A Land-use regression model. Environmental Pollution, 208 (A): 241-248.
- Sider, T., G. Goulet-Langlois, N. Eluru, M. Hatzopoulou. 2015. Evaluating the sensitivity of transport emission inventories to the level of input aggregation and model randomness. Transportation, 43:315-335.
2015
- Farrell, W., S. Weichenthal, M. Goldberg, M. Hatzopoulou. 2015. Evaluating air pollution exposures across cycling infrastructure types: Implications for facility design. Journal of Transportation and Land-Use, 8 (3): 131-149.
- Sider, T., M. Hatzopoulou, N. Eluru, G. Goulet-Langlois, K. Manaugh. 2015. Smog and socio-economics: An evaluation of equity in traffic-related air pollution exposure and generation. Environment and Planning B, 42: 870-887.
- Farrell, W., L. Deville-Cavellin, S. Weichenthal, M. Goldberg, M. Hatzopoulou. 2015. Capturing the urban canyon effect on particle number concentrations across a large road network using spatial analysis tools. Building and Environment, 92: 328 -334.
- Shekarrizfard, M., Y. Shamsunnahar, M.F. Valois, M. Goldberg, D. Crouse, N. Ross, M.E. Parent, M. Hatzopoulou. 2015. Investigating the role of transport models in epidemiologic studies of traffic related air pollution and health effects. Environmental Research, 140: 282-291.
- Dale, LM., S. Goudreau, S. Perron, MS. Ragettli, M. Hatzopoulou, A. Smargiassi. 2015. Socioeconomic status and environmental noise exposure in Montreal, Canada. BMC Public Health, 15: 205.
- Ghafghazi, G. and M. Hatzopoulou. 2015. Simulating the air quality impacts of traffic calming schemes in a dense urban neighbourhood. Transportation Research Part D, 35: 11-22.
2014
- Sider, T., A. Alam, W. Farrell, M. Hatzopoulou, N. Eluru. 2014. Evaluating vehicular emissions with an integrated mesoscopic and microscopic traffic simulation. Canadian Journal of Civil Engineering, 41 (10): 856-868
- Alam, A., G. Ghafghazi, M. Hatzopoulou. 2014. Traffic Emissions and Air Quality Near Roads in Dense Urban Neighborhood: Using Microscopic Simulation for Evaluating Effects of Vehicle Fleet, Travel Demand, and Road Network Changes. Transportation Research Record, No. 2427, pp. 83-92.
- Weichenthal, S., M. Hatzopoulou, MS. Goldberg. 2014. Impact of short-term exposure to traffic-related air pollution during physical activity on blood pressure, autonomic and micro-vascular function in women. Particle and Fiber Toxicology, 11: 70
- Alam, A., E, Diab, A. El-Geneidy, M. Hatzopoulou. 2014. A simulation of transit bus emissions along an urban corridor: Evaluating changes across several years and under various service improvement strategies. Transportation Research Part D, 31: 189-198.
- Weichenthal, S., W. Farrell, L. Joseph, M. Goldberg, M. Hatzopoulou. 2014. Characterizing the impact of traffic and built environment on near-road ultrafine particle concentrations. Environmental Research, 132: 305-310.
- Alam, A. and M. Hatzopoulou. 2014. Investigating the isolated and combined effects of congestion, roadway grade, passenger loading, and alternative fuels on transit bus emissions. Transportation Research Part D, 29: 12-21.
- Alam, A. and M. Hatzopoulou. 2014. Reducing transit bus emissions: Alternative fuels or traffic operations? Atmospheric Environment, 89: 129-139.
- Ghafghazi, G., M. Hatzopoulou. 2014. Simulating the environmental effects of isolated and area-wide traffic calming schemes using traffic simulation and microscopic emission modeling. Transportation, 41 (3): 633-649.
2013
- Sider, T., A. Alam, M. Zukari, H. Dugum, N. Goldstein, N. Eluru, M. Hatzopoulou. 2013. Land-use and socio-economics as determinants of traffic emissions and individual exposure to air pollution. Journal of Transport Geography, 33: 230-239.
- Hatzopoulou, M., S. Weichenthal, G. Barreau, M. Goldberg, W. Farrell, D. Crouse, N. Ross. 2013. A web-based route planning tool to reduce cyclists’ exposures to traffic pollution: A case study in Montreal, Canada. Environmental Research, 123: 58-61.
- Chan, L., Miranda-Moreno, Alam, A., M. Hatzopoulou. 2013. Assessing the impact of bus technology on greenhouse gas emissions along a major corridor: A lifecycle analysis. Transportation Research Part D, 20: 7-11.
- Mathez, A., K. Manaugh, V. Chakour, A. El-Geneidy, M. Hatzopoulou. 2013. How can we alter our carbon footprint? Estimating GHG emissions based on travel survey information. Transportation, 40 (1): 131-149.
- Hatzopoulou, M., S. Weichenthal, H. Dugum, G. Pickett, L. Miranda-Moreno, R. Kulka, R. Andersen, M. Goldberg. 2013. The impact of traffic counts and separate cycling lanes on personal air pollution exposures among cyclists in Montreal, Canada. Journal of Exposure Science and Environmental Epidemiology, 23 (1): 46-51.
2012
- Strauss, J., L. Miranda-Moreno, D. Crouse, M. Goldberg, Ross, N., M. Hatzopoulou. 2012. Investigating the link between cyclist volumes and pollution levels along bicycle facilities in a dense urban core. Transportation Research Part D, 17 (8): 619-625.
- Lau, J., M. Hatzopoulou, M. Wahba, E.J. Miller. 2012. An integrated multi-model evaluation of transit bus emissions in Toronto. Transportation Research Record, No. 2216, pp. 1-9.
2011
- Hatzopoulou, M., J.Y., Hao, and E.J. Miller. 2011. Simulating the impacts of household travel on greenhouse gas emissions, urban air quality, and population exposure. Transportation, 38 (6): 871-887.
2010
- Hao, J.Y., M. Hatzopoulou, and E.J. Miller. 2010. Integrating an activity-based travel demand model with dynamic traffic assignment and emission models: An implementation in the Greater Toronto Area. Transportation Research Record, No. 2176, pp. 1-13. Received the Charley V. Wootan Award for the Best Paper in Transportation Policy and Organization.
- Hatzopoulou, M. and E.J. Miller. 2010. Linking an activity-based travel demand model with traffic emission and dispersion models: Transport’s contribution to air pollution in Toronto. Transportation Research Part D, 15 (6): 315-325.
2009
- Hatzopoulou, M. and E.J. Miller. 2009. Transport policy evaluation in metropolitan areas: The role of modelling in decision-making. Transportation Research Part A, 43 (4): 323-338.
2008
- Hatzopoulou, M. and E.J. Miller. 2008. Institutional integration for sustainable transportation policy in Canada. Transport Policy, 15 (3): 149–162.
2007
- Hatzopoulou, M., E.J. Miller and B. Santos. 2007. Integrating vehicle emission modelling with activity-based travel demand modelling: A case study of the Greater Toronto Area (GTA), Transportation Research Record, No. 2011, pp. 29-39.
- Kazopoulo M., I. Kaysi and M. El-Fadel. 2007. A stated-preference approach towards assessing a vehicle inspection and maintenance program. Transportation Research Part D, 12 (5): 358-370.
2001-2005
- Kazopoulo M., M. El-Fadel and I. Kaysi. 2005. Emission standards development with exhaust emission measurements in a typical inspection and maintenance program. ASCE Journal of Environmental Engineering, 131 (9): 1330-1339.
- El Fadel, M., I. Alameddine, M. Kazopoulo, M. Hamdan and R. Nasrallah. 2001. Indoor air quality assessment in an underground parking facility. Indoor + Built Environment, 10 (3-4): 179-184.
- El Fadel, M., I. Alameddine, M. Kazopoulo, M. Hamdan and R. Nasrallah. 2001. Carbon Monoxide and Volatile Organic Compounds as indicators of indoor air quality in underground parking facilities. Indoor + Built Environment, 10 (2): 70-82.
- Tabbal, M., M. Kazopoulo, T.C. Christidis and S. Isber. 2001. Enhancement of the molecular nitrogen dissociation levels by Argon dilution in surface wave sustained plasmas. Applied Physics Letters, 78: 2131.
- Chowdhury, T., J. Vaughan, M. Saleh, K. Mousavi, M. Hatzopoulou, M. Roorda. 2022. Modeling Impacts of Off-peak Delivery in the Greater Toronto and Hamilton Area, 101st Annual Meeting of the Transportation Research Board, Washington, DC, January.
- Ahmed, U., R. Tu, J. Xu, G. Amirjamshidi, M. Hatzopoulou, M. Roorda. 2022. GPS Based Traffic Condition Classification using Machine Learning Approaches. 101st Annual Meeting of the Transportation Research Board, Washington, DC, January.
- Xu, J., R. Tu, U. Ahmed, G. Amirjamshidi, M. Hatzopoulou, M.J. Roorda. 2021. A Scoring System to Evaluate Eco-Driving Using Driving Behaviour and Vehicle Characteristics for Usage-based Insurance. 100th Annual Meeting of the Transportation Research Board, Washington, DC, January.
- Wang, A., R. Tu, J. Xu, Z. Zhai, M. Hatzopoulou. 2021. A Novel Modal Emission Modeling Approach and its Application with On-Road Emission Measurements. 100th Annual Meeting of the Transportation Research Board, Washington, DC, January.
- Tu, R., A. Wang, J. Xu, Z. Zhai, M. Hatzopoulou. 2021. Real World Emissions under Local Drive Cycles and Comparison with Certification Testing. 100th Annual Meeting of the Transportation Research Board, Washington, DC, January.
- Djavadian, S., L. Alfaseeh, R. Tu, B. Farooq, M. Hatzopoulou. 2020. Multi-Objective Eco-Routing in a Distributed Traffic Management Framework with a Case Study of Downtown Toronto. 99th Annual Meeting of the Transportation Research Board, Washington, DC, January.
- Tu, R., Y. Gai, D. Posen, B. Farooq, M. Hatzopoulou. 2020. Electric Vehicle Charging Optimization to Minimize Marginal Greenhouse Gas Emissions. 99th Annual Meeting of the Transportation Research Board, Washington, DC, January.
- Xu J., A. Wang, N. Schmidt, M. Adams, M. Hatzopoulou. 2020. A Machine Learning Approach for Predicting Near-Road Ultrafine Particle Concentrations Using Local Traffic Characteristics. 99th Annual Meeting of the Transportation Research Board, Washington, DC, January.
- Xu J., A. Wang, R. Tu, M. Saleh, N. Schmidt, M. Hatzopoulou. 2020. Data-Driven Approach to Capture the Association Between Local Truck Movements and Near-Road Black Carbon Concentrations Using Mobile Measurements. 99th Annual Meeting of the Transportation Research Board, Washington, DC, January.
- Xu J., M. Saleh, A. Wang, R. Tu, M. Hatzopoulou. 2019. Towards a Canadian Version of the MOVES Model: Capturing Driving Behaviours in Greater Toronto and Comparison against US Defaults. 98th Annual Meeting of the Transportation Research Board, Washington, DC, January.
- Xu J., M. Saleh, M. Hatzopoulou. 2019. A Machine Learning Approach Capturing the Effects of Meteorology, Time of Day, Driving Behaviour, and Driver Experience on Trip-Level Emissions. 98th Annual Meeting of the Transportation Research Board, Washington, DC, January.
- Tu R., L. Alfaseeh., S. Djavadian., M. Saleh., B. Farooq., M. Hatzopoulou. 2019. What Happens to On-Road Emissions when Travel Time on a Road Network is Improved Through End-to-End Dynamic Routing for Connected Autonomous Vehicles? 98th Annual Meeting of the Transportation Research Board, Washington, DC, January.
- Tu R., A. Wang, M. Hatzopoulou. 2019. Improving the Spatial Accuracy of Regional Emission Inventories and Investigating the Transferability of Emission Modeling Approaches across Different Cities. 98th Annual Meeting of the Transportation Research Board, Washington, DC, January.
- Wang, A., R. Tu, Y. Gai, L. Pereira, I.D. Posen, M. Hatzopoulou. 2019. Capturing the Uncertainties in Regional Emission Estimates Related to Vehicle Electrification Can Improve the Robustness of Decision-Making. 98th Annual Meeting of the Transportation Research Board, Washington, DC, January.
- Gai, Y., A. Wang, M. Hatzopoulou, I.D. Posen. 2019. Marginal Greenhouse Gas Emissions of Ontario's Electricity System and the Implication of Electric Vehicle Charging. 98th Annual Meeting of the Transportation Research Board, Washington, DC, January.
- Reddy B.C., A. Ganji, S. Abiola, M. Hatzopoulou. 2019. Analyzing the Effects of Transit-only Corridors on Traffic and Emissions in the Affected Neighbourhoods: A Before-After Intervention Analysis. 98th Annual Meeting of the Transportation Research Board, Washington DC, January.
- Talaat, H., H. Abdel-Gawad. 2018. Mobile monitoring of Black Carbon levels and development of exposure surfaces reflecting traffic-related air pollution: The case of cairo. 98th Annual Meeting of the Transportation Research Board, Washington, DC, January.
- Shekarrizfard, M., L. Minet, M. Fallah-Shorshani, M. Hatzopoulou. 2018. Enhancing public health assessment by incorporating daily activities in the analysis of population exposure to traffic-related air pollution. 97th Annual Meeting of the Transportation Research Board, Washington, DC, January.
- Minet, L., R. Liu, M.F. Valois, J. Xu, S. Weichenthal, M. Hatzopoulou. 2018 A comparison of air pollution exposure surfaces developed via mobile and fixed-site monitoring protocols against personal exposures. 97th Annual Meeting of the Transportation Research Board, Washington, DC, January.
- Minet, L., J. Stokes, J. Scott, M.F. Valois, J. Xu, S. Weichenthal, M. Hatzopoulou. 2018. Should traffic-related air pollution be considered when designing urban bicycle networks? 97th Annual Meeting of the Transportation Research Board, Washington, DC, January.
- Liu, R., L. Minet, M. Shekarrizfard, M. Fallah-Shorshani, S. Weichenthal, M. Hatzopoulou. 2018. Short-term exposure to ultrafine particles, black carbon and noise: Comparison between panel data and mobility-based exposures derived from land-use regression models. 97th Annual Meeting of the Transportation Research Board, Washington, DC, January.
- Tu, R., I. Kamel, B. Abdulhai, M. Hatzopoulou. 2018. Reducing transportation greenhouse gas emissions through the development of policies targeting high-emitting trips. 97th Annual Meeting of the Transportation Research Board, Washington, DC, January.
- Tu, R., I. Kamel, A. Wang, B. Abdulhai, M. Hatzopoulou. 2018. Developing policy-sensitive transportation GHG emission inventories: Which model resolution and input detail is appropriate? 97th Annual Meeting of the Transportation Research Board, Washington, DC, January.
- Xu, J., R. Tu, A. Wang, L. Minet, C. Stogios, M. Saleh, N. Hilker, J. Wang, G. Evans, M. Hatzopoulou. 2018. Quantifying the contribution of diesel vehicles to traffic emissions along an urban corridor: Implications for cleaner public transit. 97th Annual Meeting of the Transportation Research Board, Washington, DC, January.
- Xu, J., J. Wang, N. Hilker, M. Fallah-Shorshani, R. Tu, A. Wang, L. Minet, C. Stogios, G. Evans, M. Hatzopoulou. 2018. Evaluation of MOVES emission factors against data from on-road measurements in a large Canadian city. 97th Annual Meeting of the Transportation Research Board, Washington, DC, January.
- Wang, A., C. Stogios, Y. Gai, J. Vaughan, G. Ozonder, S. Lee, D.I, Posen, E.J. Miller. M. Hatzopoulou. 2018. Automated, electric, or both? Investigating the effects of transportation and technology scenarios on metropolitan greenhouse gas emissions. 97th Annual Meeting of the Transportation Research Board, Washington, DC, January.
- Stogios, C., M. Saleh, A. Gangi, R. Tu, J. Xu, M. Roorda, M. Hatzopoulou. 2018. Determining the effects of automated vehicle driving behavior on vehicle emissions and performance of an urban corridor. 97th Annual Meeting of the Transportation Research Board, Washington, DC, January.
- Fallah-Shorshani, L. Minet, R. Liu, C. Plante, S. Goudreau, A. Smargiassi, S. Weichenthal, M. Hatzopoulou. 2018. Capturing the spatial variability of noise levels using land use regression models and comparing noise surfaces against personal exposures collected through a panel study. 97th Annual Meeting of the Transportation Research Board, Washington, DC, January.
- Kumar Dey, B., S. Anowar, N. Eluru, M. Hatzopoulou. 2018. Accommodating exogenous variable and decision rule heterogeneity in discrete choice models: Application to bicyclist route choice. 97th Annual Meeting of the Transportation Research Board, Washington, DC, January.
- Addo, A. and M. Hatzopoulou. 2017. Harnessing the potential of AVL and APC data to simulate greenhouse gas emissions of interregional bus route in Toronto, Canada. 96th Annual Meeting of the Transportation Research Board, Washington, DC, January
- Anowar, S., N. Eluru, M. Hatzopoulou. 2017. Quantifying the value of a clean ride: How far would you bicycle to avoid exposure to traffic-related air pollution? 96th Annual Meeting of the Transportation Research Board, Washington, DC, January
- Anowar, S., N. Eluru, M. Hatzopoulou. 2017. Who are commuter and non-commuter cyclists? An in-depth exploration of their characteristics, habits and perceptions. 96th Annual Meeting of the Transportation Research Board, Washington, DC, January
- Fallah-Shorshani, M., M. Shekarrizfard, M. Hatzopoulou. 2017. Integrating a street-canyon model with regional Gaussian dispersion model for improved characterization of near-road air pollution. 96th Annual Meeting of the Transportation Research Board, Washington, DC, January
- Addo, A. and M. Hatzopoulou. 2017. Daily variations in ultrafine particle concentrations at intersections versus midblock locations along a busy transit corridor. 96th Annual Meeting of the Transportation Research Board, Washington, DC, January
- Xu, J., M. Turchet, N. Hilker, M. Fallah-Shorshani, G. Evans, M. Hatzopoulou. 2017. Contrasting direct use of data from traffic radar and video cameras with traffic simulation software in estimation of vehicle emissions. 96th Annual Meeting of the Transportation Research Board, Washington, DC, January
- Xu, J., M. Hatzopoulou. 2016. Investigating effects of meteorology, built environment, and traffic composition on near-road air pollution in dense urban areas. 95th Annual Meeting of the Transportation Research Board, Washington, DC, January
- Shekarrizfard, M., M. Hatzopoulou. 2016. Development and validation of an exposure surface for traffic related air pollution using dispersion modelling against land use regression. 95th Annual Meeting of the Transportation Research Board, Washington, DC, January.
- Widener, M., M. Hatzopoulou. 2016. Contextualizing research on transportation and health: Systems perspective. 95th Annual Meeting of the Transportation Research Board, Washington, DC, January.
- Waraich, A., A. Sabreena, T. Tenaglia, T. Sider, A. Alam, N. Shaabanzaadeh Minaei, M. Hatzopoulou, N. Eluru. 2016. Disaggregate-Level Simulation of Public Transit Emissions in a Large Urban Region. 95th Annual Meeting of the Transportation Research Board, Washington, DC, January.
- Deville Cavellin, L., S. Weichenthal, R. Tack, M. Ragettli, A. Smargiassi, M. Hatzopoulou. 2016. Investigating the use of portable air pollution sensors to capture spatial variability of traffic-related air pollution. 95th Annual Meeting of the Transportation Research Board, Washington, DC, January.
- Shekarrizfard, M., A. Alam, A. Badran, J. Faucher, L. Miranda-Moreno, C. Morency, N. Saunier, Z. Patterson, M. Trepanier. 2016. Validation of a PUFF dispersion model: Air quality simulation for new highway infrastructure. 95th Annual Meeting of the Transportation Research Board, Washington, DC, January.
- Alam, A. and M. Hatzopoulou. 2015. An analysis of instantaneous speed distributions and emissions for transit buses across an urban network. Proceedings of the 94th Annual Meeting of the Transportation Research Board, Washington, DC, January.
- Alam, A. and M. Hatzopoulou. 2015. Modeling Transit bus emissions using MOVES: Validation of default distributions and embedded drive cycles with local data. Proceedings of the 94th Annual Meeting of the Transportation Research Board, Washington, DC, January.
- Sazavar, A., M. Hatzopoulou, M. Roorda. 2015. A peer-to-peer matching system for grocery home delivery. Proceedings of the 94th Annual Meeting of the Transportation Research Board, Washington, DC, January.
- Farrell, W., L. Deville-Cavellin, S. Weichenthal, M. Goldberg, M. Hatzopoulou. 2015. Capturing the urban canyon effect on near-road air pollution concentrations. Proceedings of the 94th Annual Meeting of the Transportation Research Board, Washington, DC, January.
- Shekarrizfard, M., A. Alam, A. Badran, J. Faucher, L. Miranda-Moreno, C. Morency, Z. Patterson, N. Saunier, M. Trépanier, M.Hatzopoulou. 2015. Validation of a puff dispersion model: Air quality simulation for new highway infrastructure. Accepted and withdrawn, 94th Annual Meeting of the Transportation Research Board, Washington, DC, January.
- Farrell, W. and M. Hatzopoulou. 2014. Evaluating air pollution exposures across cycling infrastructure types: Implications for facility design. 2014 World Symposium on Transportation and Land Use research, Delft, the Netherlands.
- Alam, A. and M. Hatzopoulou. 2014. Quantifying the influencing factors of transit bus emissions and evaluating the potential of service improvements and alternative fuels on emission reductions. Proceedings of the 2014 Transportation Land Use Planning and Air Quality Conference, North Carolina.
- Diab, E. and M. Hatzopoulou. 2014. Investigating the air pollution impacts of major events: A study of the effects of Montreal’s largest festivals on downtown air quality. Proceedings of the 2014 Transportation Land Use Planning and Air Quality Conference, North Carolina.
- Alam, A., E, Diab, A. El-Geneidy, M. Hatzopoulou. 2014. Investigating the impacts of service improvement strategies on transit bus emissions along a busy urban corridor. Proceedings of the 93rd Annual Meeting of the Transportation Research Board, Washington, DC, January.
- Farrell, W., S. Weichenthal, M. Goldberg, M. Hatzopoulou. 2014. A statistical model explaining air pollution exposures of cyclists in urban environments. Proceedings of the 93rd Annual Meeting of the Transportation Research Board, Washington, DC, January.
- Ghafghazi, G. and M. Hatzopoulou. 2014. Simulating the air quality impacts of traffic calming schemes in a dense urban neighbourhood. Proceedings of the 93rd Annual Meeting of the Transportation Research Board, Washington, DC, January.
- Sider, T., M. Hatzopoulou, N. Eluru, G. Goulet-Langlois, K. Manaugh. 2014. Smog and socio-economics: An evaluation of equity in traffic-related air pollution exposure and generation. Proceedings of the 93rd Annual Meeting of the Transportation Research Board, Washington, DC, January.
- Ghafghazi, G. and M. Hatzopoulou. 2013. Simulating the environmental effects of isolated and network-wide traffic calming schemes using traffic simulation and microscopic emission modeling. Proceedings of the 92nd Annual Meeting of the Transportation Research Board, Washington, DC, January.
- Chan. S., A. Alam, L. Miranda-Moreno, M. Hatzopoulou. 2013. Assessing the impact of bus technology on GHG emissions along a major corridor: comparing an instantaneous speed emission model with an average-speed model. Proceedings of the 92nd Annual Meeting of the Transportation Research Board, Washington, DC, January.
- Farrell, W., S. Weichenthal, M. Goldberg, N. Brownlie, B. Moulins, J. Neves-Pelchat, G. Pickett, R. Kim, R. Luck, TH. Truong, M. Zukari, M. Hatzopoulou. 2013. Measuring cyclists’ exposure to vehicle induced emissions across regional cycling facilities. Proceedings of the 92nd Annual Meeting of the Transportation Research Board, Washington, DC, January.
- Sider, T., A. Alam, M. Zukari, H. Dugum, N. Goldstein, N. Eluru, M. Hatzopoulou. 2013. Quantifying the effects of land-use and socio-economics on the generation of traffic emissions and individual exposure to air pollution at a metropolitan scale. Proceedings of the 92nd Annual Meeting of the Transportation Research Board, Washington, DC, January.
- Alam, A. And M. Hatzopoulou. 2013. A microscopic simulation of transit bus emissions under transit signal priority and alternative technology. Proceedings of the 92nd Annual Meeting of the Transportation Research Board, Washington, DC, January.
- Chan. S., A. Alam, L. Miranda-Moreno, M. Hatzopoulou. 2012. Investigating the effect of technology and operation on lifecycle greenhouse gas emissions for bus service in Montreal. Proceedings of the 12th International Conference on Advanced Systems for Public Transport (CASPT12), Santiago, Chile, July.
- Strauss, J., L. Miranda-Moreno, D. Crouse, M. Goldberg, M. Hatzopoulou. 2012. Investigating the link between cyclist volumes and pollution levels along bicycle facilities in a dense urban core. Proceedings of the 91st Annual Meeting of the Transportation Research Board, Washington, DC, January.
- Mathez, A., K. Manaugh, V. Chakour, A. El-Geneidy, M. Hatzopoulou. 2012. How can we alter our carbon footprint? Estimating GHG emissions based on travel survey information. Proceedings of the 91st Annual Meeting of the Transportation Research Board, Washington, DC, January.
- Hatzopoulou, M., E.J. Miller, and J.Y. Hao. 2009. Linking an activity-based travel demand model with emission, dispersion, and population exposure models for appraisal of strategic transport plans. Proceedings of the 12th Conference of the International Association for Travel Behaviour Research (IATBR), Jaipur, India, December.
- Hatzopoulou, M. and E.J. Miller. 2008. Sustainable transportation in Canadian metropolitan areas: Assessing the mechanisms for planning, funding, and implementation. Proceedings of the 2008 Conference of the Transportation Association of Canada, Toronto, September.
- Hatzopoulou, M., B. Santos and E.J. Miller. 2008. Developing regional 24-hour profiles for link-based, speed-dependent vehicle emissions and zone-based soaks. CD Proceedings of the 87th Annual Meeting of the Transportation Research Board, Washington, DC, January.
- Hatzopoulou, M. and E.J. Miller. 2008. Sustainable transportation: Theory or practice? A perspective of planners and policy-makers. CD Proceedings of the 87th Annual Meeting of the Transportation Research Board, Washington, DC, January.
- Hatzopoulou, M. and E.J. Miller. 2007. Appraisal of transport policy and strategic plans in Canadian urban areas: A survey of planners and policy-makers in government agencies across Canada. CD Proceedings of the 11th World Conference on Transportation Research, Berkeley, CA, June.
- Hatzopoulou, M. and E.J. Miller. 2006. Indicators of sustainable transport for integrated policy appraisal: Linking sustainable transport plans with decision-making in the Greater Toronto Area (GTA). CD Proceedings of the New Scholars Conference on Sustainable Transportation, Bloomington, Indiana, May.
- Buliung, R.N., M. Hatzopoulou and M. Roorda. 2006. Plugging the “environment” into Integrated Urban Models: Recent progress and research direction. Proceedings of the Canadian Transportation Research Forum, Quebec City, Quebec, May.
- Kazopoulo, M., I. Kaysi and M. El-Fadel. 2004. Implementing an inspection and maintenance program: A stated-preference approach. CD Proceedings of the 10th World Conference on Transport Research, Istanbul, Turkey, July.
- Tabbal, M, T. Christidis, S. Isber and M. Kazopoulo. 2001. Nitrogen atom production in N2, Ar/N2 and He/N2 surface wave discharges. 15th International Symposium on Plasma Chemistry, 6: 2237, Orleans, France, July.
- Kazopoulo, M., M. Tabbal, T. Christidis and S. Isber. 2001. Characterization of a surface wave discharge for the deposition of nitride thin films. Proceedings of the Second French Lebanese Colloquium on Materials Sciences (CSM2), Beirut, Lebanon, May.
Group
Sabreena Anowar, 2016-2018
Maryam Shekarrizfard, 2016-2018
Masoud Fallah-Shorshani, 2014-2017
Ran Tu, 2020
Laura Minet, 2020
Zhiqiang Zhai (2018-2020)
An Wang (2019-2021)
Junshi Xu (2020-2023)
PhD | Thesis Title | Completion |
Wang, A. | Extending the boundaries of emission and dispersion modelling with uncertainty analysis and data-driven models | Oct-20 |
Minet, L. | From bike lanes to highway trucks, assessing the impact of transportation policies on air pollution exposure at local and regional scales | Jun-20 |
Tu, R. | Traffic emission modelling for robust policy design in connected and electric transportation | Apr-20 |
Xu, J. | Individual and environmental determinants of traffic emissions and near-road air quality | Jan-20 |
Shekarrizfard, M. | Investigating population exposure to traffic-related nitrogen oxides through the development and validation of air pollution surfaces | May-16 |
Alam, A. | Capturing the effects of urban drive cycles and passenger ridership on transit bus emissions and investigating the potential of emission reduction strategies | Aug-15 |
MSc-Thesis | Thesis Title | Completion |
Mallinen, K. | Impact of rail emissions on neighbourhood air pollution exposure | Dec-21 |
Gai, Y. | Lifecycle emissions of long-range vehicle fleet scenarios in the Greater Toronto Area | Apr-19 |
Stogios, C. | Modelling the impact of automated vehicles on traffic emissions | Apr-18 |
Wang, A. | Characterizing near-road air pollution using local-scale emission and dispersion models and validation against in-situ measurements | May-16 |
Minet, L. | Testing the robustness of land-use regression models for atmospheric nitrogen dioxide and ozone using data from fixed and mobile monitoring campaigns | May-16 |
Lee, A. | Influential parameters of near-roadway ultrafine particles along open patio spaces | Dec. 2015 |
Deville-Cavellin, L. | Investigating the use of portable air pollution sensors to capture the spatial variability of traffic related air pollution | Aug. 2015 |
Xu, J. | Investigating the effects of meteorology, built environment and traffic on near road air pollution | Aug. 2015 |
Sazavar, A. | A peer-to-peer matching system for grocery home delivery | Sept. 2014 |
Farrel, W. | Understanding the effects of the urban environment on cyclist exposure to near-roadway air pollution | Sept. 2014 |
Sider, T. | Development of an integrated transport and emissions model and applications for population exposure and environmental justice | Dec. 2013 |
Ghafghazi, G. | Investigating the effects of traffic calming on near-road air quality using traffic, emissions, and air dispersion modelling | Sept. 2013 |
MEng-Project | Thesis Title (or general topic) | Completion |
Gong, J. | Traffic characterization using Streetview images | Apr-22 |
Abiola, S. | Impacts of freight traffic on regional air quality | Apr-19 |
Channa-Reddy, B. | Traffic and emission simulation for the King Street pilot project | Dec-18 |
Dean, K. | Modelling ground level PM2.5 concentrations from traffic emissions in downtown Toronto | Dec-17 |
Farhad, M. | Dispersion modelling for highway 25 in Montreal | Aug-17 |
Stokes, J. | Development of land-use regression models based on mobile air pollution data collected along bicycle facilities | Aug-16 |
Undergraduate Fellow | Research Project | Duration |
Kang, J. | Traffic characterization using Streetview images | Summer 2021 |
Sok, P. | Capturing the effect of land-use and the built environment on traffic-related air quality in large metropolitan areas | Summer 2017 |
Moreaux, J. | Capturing the effect of land-use and the built environment on traffic-related air quality in large metropolitan areas | Summer 2017 |
Liu, R. | Processing of individual mobility data and air pollution exposure | Summer 2017 |
Tu, X. | Data collection and mapping of near-road air quality in Toronto | Summer 2016 |
Wint, M.S | Data collection and mapping of near-road air quality in Toronto | Summer 2016 |
Scott, J. | Data collection and mapping of near-road air quality in Toronto | Summer 2016 |
Kuruparan, S | Data collection and mapping of near-road air quality in Toronto | Summer 2016 |
Chow, T. | Data collection and mapping of near-road air quality in Toronto | Summer 2016 |
Kang, T. | Data collection and mapping of near-road air quality in Toronto | Summer 2016 |
Mario, J. | Data collection and mapping of near-road air quality in Toronto | Summer 2016 |
Li, J. | Data collection and mapping of near-road air quality in Toronto | Summer 2016 |
Sun, S. | Panel study of air pollution exposure and cardiovascular health | Summer 2016 |
Turchet, M. | Microsimulation of traffic emissions on College Street | Summer 2016 |
Bridi, P. | Mobile sensing of near-road air pollution | Summer 2015 |
Lueders, B. | Mobile sensing of near-road air pollution | Summer 2015 |
Nahed, K. | Mobile sensing of near-road air pollution | Summer 2015 |
Jaber, A. | GPS data collection on-board transit buses | Winter 2015 |
Wai, J. | Air pollution monitoring in Montreal | Summer 2014 |
Tack, R. | Testing the performance of air pollution micro-sensors | Winter 2014 |
Summer 2014 | ||
Fall 2014 | ||
Summer 2015 | ||
Crohmal, G. | Air pollution monitoring in Montreal | Summer 2014 |
Addo, A. | Analysis of bus transit emissions in Montreal | Winter 2014 |
Diamond, K. | Air pollution and cardiovascular effects among women cyclists | Summer 2013 |
Ozols-Mongeau, B. | Air pollution and cardiovascular effects among women cyclists | Summer 2013 |
Mathewson, E. | Air pollution and cardiovascular effects among women cyclists | Summer 2013 |
Olcusenler, M. | Air pollution monitoring in urban microenvironments | Summer 2013 |
Asthana, S. | Quantifying transit bus emissions in Montreal | Summer 2013 |
Reda, W. | Quantifying transit buses emissions in Montreal | Summer 2013 |
Fall 2013 | ||
Hanson, S. | UFP sampling campaign in Montreal region | Winter 2013 |
Goulet-Langlois, G. | Modelling start and soak emissions of passenger vehicles | Winter 2013 |
Moulins, B. | Measuring cyclists’ exposure to air pollution | Summer 2012 |
Brownlie, M. | Measuring cyclists’ exposure to air pollution | Summer 2012 |
Neves-Pelchat, J | Measuring cyclists’ exposure to air pollution | Summer 2012 |
Summer 2013 | ||
Luck, R. | Measuring cyclists’ exposure to air pollution | Summer 2012 |
Truong, T. | Measuring cyclists’ exposure to air pollution | Summer 2012 |
Pickett, G. | Measuring cyclists’ exposure to air pollution | Summer 2011- |
Winter 2013 | ||
Zukari, M. | Developing a travel emissions footprint on a household basis | Fall 2011 |
Fall 2012 | ||
Winter 2013 | ||
Goldstein, N. | Modelling the interaction between vehicle ownership and land-use | Summer 2011 |
Fall 2011 | ||
Winter 2012 | ||
Dugum, H | Modelling the interaction between vehicle ownership and land-use | Summer 2011 |
Fall 201 | ||
Winter 2012 | ||
Somos, A. | Measuring cyclists’ exposure to air pollution | Summer 2011 |
Mathez, A. | Calculating the Carbon Footprint of Commuting to McGill | Summer 2011 |
Chakour, V. | McGill Transport Survey | Summer 2011 |
Khattab, M. | Modelling the Dispersion of Road Emissions in Lisbon | Summer 2010 |
Lau, J. | An Integrated Evaluation of Transit Bus Emissions in Toronto | Fall 2008 |
Winter 2009 | ||
NSERC USRA | Research Project | Duration |
Daw, E. | Air quality in Bathurst Quay neighborhood | Summer 2022 |
Zamel Y. | Vehicle characterization using street view images | Summer 2021 |
Gusz, C. | Monitoring of ambient fine particles in Toronto | Summer 2018 |
Liu, R. | Processing of individual mobility data and air pollution exposure | Summer 2017 |
Dale, J. | Panel study relating air pollution exposure to cardiovascular outcomes in pedestrians and cyclists | Summer 2016 |
Thesis Abstracts for TRAQ Graduates (MASc and PhD)
The transportation system is considered as a prominent source of greenhouse gases (GHG) and air pollution in urban areas. Urban transportation systems are undergoing revolutionary changes, including vehicle automation and electrification, and their environmental impacts remain uncertain. Can existing emission and air quality modelling techniques adequately perform in the face of these drastic changes? How can we extend the capabilities of current physical emission models and empirical air quality models? In this thesis, we addressed these questions focusing on two themes: 1) regional traffic emission inventories; 2) data-driven models for local emission and air quality characterization.
In theme 1, we first developed a modelling approach to generate a regional emission inventory of passenger transportation (public transit and private household vehicle trips) in the Greater Toronto and Hamilton Area (GTHA). The model was also used to assess the fuel cycle emissions from automated and electric vehicles (AV & EV). When including only public transit and private household vehicle trips, the latter contributes 96% of regional GHG emissions for passenger transportation. With the introduction of AVs, additional private household vehicle kilometres are expected. Moreover, the GHG emission reduction potential of EVs is highly dependent on the GHG emissions of the local power grid. We identified various sources of uncertainty in current vehicular emission models and quantified these sources of uncertainty with a Monte-Carlo approach. Using data for the GTHA, we investigated the combined effects of vehicle electrification and fuel cycle emission uncertainty. Vehicle operating emissions dominated the total GHG emissions and dominated the uncertainty from other sources. The introduction of EVs also reduced emission uncertainty, but at a low penetration rate, their GHG emission reduction potential can be less effective compared to eco-driving and congestion reduction.
Theme 2 focuses on local scale emission and air quality modelling. Noticing the importance of uncertainty in operating emissions, we proposed a novel modal emission modelling approach for vehicle hot stabilized running emissions, based on measured emissions. The approach treats the emission data stratification as an optimization problem and solves the problem based on the Greedy algorithm to define the boundaries of emission strata. This approach can effectively reproduce emissions in steady and aggressive driving conditions. Our approach can effectively balance between computational complexity and accuracy by tuning the resolution of explanatory variables. The last part of this thesis explores the use of a traditional empirical air quality model (land use regression (LUR)) compared to novel machine learning techniques (neural network and gradient boost). Both empirical models were applied to air quality data collected during a mobile sampling campaign in downtown Toronto. We compared their performance and explored their application boundaries by contrasting how explanatory variables were expressed in LUR and machine learning models. We concluded that machine learning could perform better with abundant data, and the prediction power of LUR was dependent on the monotonic relationship between explanatory and response variables.
In a context of increasing concern for population exposure to ambient air pollution, it is crucial to develop tools to assist urban policies tackling air quality. In this thesis, techniques to investigate air quality at local and regional scales were developed and applied to analyze the impact of transportation policies on population exposure and health.
The first part of this thesis consists in a large data collection campaign involving short-term stationary and mobile measurements of air pollutant concentrations in Toronto. Land-use regression (LUR) models based on the two data collection protocols are developed and compared with data from a panel study. Recommendations are provided for the design of short-term monitoring campaigns.
In the second part of this thesis, we set-up a chemical transport model (CTM) and a plume-in-grid (PinG) model for the Greater Toronto and Hamilton Area (GTHA) to simulate the levels of various criteria air contaminants at a scale of 1 km2. These models are based on a detailed traffic emission inventory and enable a refined analysis of population exposure to traffic-related air pollution. The CTM is combined with a health impact assessment tool to investigate the benefits of greening transport freight movements on urban air quality and health.
In the third module, we use our LUR and air quality models to analyze the impact of transportation policies on population exposure and health. The fine spatial resolution of the LUR exposure surfaces is optimal to assess a major urban planning strategy of the City of Toronto: the installation of new bike facilities. We use our CTM to investigate the health co-benefits of greenhouse gas (GHG) mitigation strategies for the transportation sector.
While we recommend the use of detailed air pollutant maps such as those obtained from LUR models to assist local policy making, spatially refined CTMs supplemented by comprehensive emission inventories are outstanding tools to assess and compare the benefits of transportation policies at a regional scale.
Traffic emissions such as greenhouse gases (GHGs, in CO2eq) and nitrogen dioxides (NOx), as well as traffic-related air pollution lead to many global problems such as climate change and public health issues. In order to mitigate the impacts on emissions and air pollution of growing urban traffic, travel demand management, driving operation control, and advanced technology initiatives have been implemented in various cities. The aim of this research is to tackle the challenge of achieving robust transportation policy decisions with accurate emission estimation approaches both at local and regional levels. In the first module of the thesis, the emission factor (EF, in grams of traffic emissions per unit distance) within one traffic condition, which is commonly defined as a single value, is expanded to a distribution. A regional emission distribution is therefore established using the EF distribution, enhancing the robustness of policy analysis. Meanwhile, the identification of the EF variation leads to the development of a hybrid machine-learning based emission estimation approach, CLustEr-based Validated Emission Re-calculation (CLEVER). The CLEVER approach is able to generate more refined traffic condition categories and corresponding representative EFs with the use of multiple resolutions of traffic data. An accurate regional emission estimation can be achieved using the CLEVER approach, while minimizing the detail needed in traffic data and the calculation burden. In the second module, several traffic emission control strategies are tested from perspectives of emissions, air pollution, and energy consumption. First, a travel demand control that specifically targets high-emitting trips is tested. Compared to a short-distance trip control, the proposed control strategy is more effective at reducing GHG emissions and decreasing total traffic delay. Second, a travel-time minimized routing algorithm with connected automated vehicles is applied in an urban road network. Potential increases of near-road nitrogen dioxide (NO2) concentrations are illustrated through investigating the impacts of the algorithm on emissions and air pollution. Lastly, regional electric vehicle charging activities are optimally scheduled with the objective of minimizing electricity generation life-cycle emissions. The optimized plan demonstrates high potential for reducing GHG emissions. However, trade-offs between emission reductions and charging facility costs are identified by comparing the optimized plan with non-optimized EV charging scenarios. This research achieves a reliable regional traffic emission estimation with much less data requirement. Based on that, innovative control strategies proposed in this research and their comprehensive evaluation process can contribute to a robust transportation policy decision.
On-road motor vehicles are responsible for a considerable proportion of near-road air pollution. While background levels of air pollutants are continuously tracked by regional monitoring networks, assessing near-road air quality remains a challenge in urban areas with complex built environments, traffic composition, and meteorological variation, leading to significant spatiotemporal variability in air pollution. This research addresses current gaps in the literature on local traffic emissions and near-road air quality.
This thesis first investigates the effect of traffic volume and speed data on the simulation of vehicle emissions and hotspot analysis. Traffic emissions are estimated using radar data as well as simulated traffic based on various speed aggregation methods. It provides recommendations for project-level analysis and particulate matter (PM) hotspot analysis.
We further compare fleet averaged emission factors (EFs) derived from a traffic emission model, the Motor Vehicle Emissions Simulator (MOVES), with EFs using plume-based measurements. This second module stresses the need to collect local traffic information for a better understanding of on-road traffic emissions. Besides, we validate default drive cycles in MOVES against representative drive cycles derived based on real-world GPS data. The validation results are helpful for transportation planners to quantify uncertainties in emission estimation and employ appropriate methods to improve the estimation of on-road emission inventories.
The third module develops eco-score models and evaluates the effect of various factors such as driver and trip characteristics on emission intensities. The results shed light on the impact of driving style on emissions and identify the most important factors affecting the amount of emissions generated by every individual driver.
The fourth module focuses on the impact of traffic emissions on near-road air quality and presents the results of two different experiments. First, it explores the effect of various factors on near-road ultrafine particle (UFP) concentrations based on short-term fixed monitoring, which stresses the significance of using local traffic characteristics to improve near-road air quality prediction. In addition it captures the distribution of truck movements in urban environments and investigates the impacts of land-use variables and detailed traffic information on near-road Black Carbon (BC) concentrations.
The transportation sector is a big source of greenhouse gas and air pollution emissions. Though studies generally agree that introducing electric vehicles (with no exhaust emissions) brings environmental benefits, an accurate assessment requires detailed analysis of emissions from electricity generation, vehicle travel patterns, and air pollutants transport.
By operationalizing the concept of marginal emission factors and simulating charging patterns using person-level travel activity data, this study developed a framework to evaluate the emission reduction potential of EV deployment in the Greater Toronto and Hamilton Area (GTHA). Then, to assess the health and economic impacts, an integrated model was built by combining a high-resolution chemical transportation model with an emission inventory with traffic and electricity generation details. According to the results, EV deployment in the GTHA can achieve significant health and climate benefits, and there is a need for policies to promote EV uptake and manage rising electricity demand.
Automated vehicles (AV) will inevitably have an impact on the movement of people and goods. Assessing the effects of AVs on land use, congestion and the environment have become of great interest to researchers. This study explores the effects of AVs and vehicle electrification on greenhouse gas emissions using traffic microsimulation and emissions modeling. The driving behaviour parameters of a traffic simulation package, most relevant to AVs, are tested within the ranges deemed to be representative of potential AV operations. The effects of AVs are evaluated under both uninterrupted and interrupted traffic flow operating environments, as well as under high and low traffic demand. The main findings indicate that automated vehicles can bring positive changes in terms of emission and traffic flow performance. The significance of the impacts is more evident when AVs are tuned to more aggressive driving settings and especially under high traffic conditions in uninterrupted flow operations.
Transport plays a crucial role in urban development by providing access to education, markets, employment, recreation, health care and other key services. Currently, 82% of Canadian commuters drive to work while the remainder rely on public transit and active transportation. In Canada, on-road traffic accounts for 19% of nitrogen oxide (NOx) emissions and in Montreal, Canada’s second largest city, transportation accounts for 85% of NOx emissions. In urban areas, NOx often refers to nitric oxide (NO) and nitrogen dioxide (NO2) since the contribution of other nitrogen oxides is minimal. NOx concentrations are often used as a marker of road traffic emissions and NOx is always higher in the vicinity of roadways and lower further away. Exposure to traffic-related air pollution has been associated with various acute and chronic respiratory and cardiovascular effects and a number of studies have established positive associations between various cancers and exposure to NO2. Most of these assessments have relied on NO2 sampling rather than modelling. Certainly, measurements alone cannot be sufficient for exposure analysis in an urban environment. As a result, there is a need for analysis tools and models that can assist policy-makers in evaluating the impacts of transport policies on urban air quality and population exposure.
This research starts with developing and validating an integrated transportation emission dispersion modelling system to be ultimately used as a tool for evaluating the potential air quality impacts of on-road transportation in the Montreal metropolitan area. We observed that simulated concentrations were reasonably correlated with the observed data when detailed meteorological and dispersion characteristics are accounted for.
The second part of this research explores the possibility of using the transportation emission model, a component of the integrated modelling system, as a proxy to a land-use regression (LUR) model for deriving exposure. We noted similarities in the risks of breast and prostate cancer associated with NO2 derived from the LUR model and NOx derived from the emission model. In the third part of the research, since travel activity, land-use patterns, and the distribution of traffic often lead to inequities in the exposure to vehicle-related air pollutants, we investigated the inequity in the emissions of -and exposure to- traffic-related air pollution, based on LUR NO2 maps and mobility information. For this purpose, long term LUR NO2 maps are used to find the daily average NO2 concentration at the home location (static), and then compared to NO2 maps (dynamic) obtained from the integrated model. We also estimated exposures during travel to identify the contribution of travelling/commuting to daily exposure. The aim of this study is to understand how individuals’ mode choices, trajectories, and activity locations affect their exposure to traffic related air pollution. In addition, the individual-level analysis allowed us to identify individuals who reduce and those who increase their exposure during their daily trajectories and activities compared to the air quality at their home location. Our results showed that individuals who live in densely populated areas may be exposed to higher concentrations while generating low levels of emissions throughout their daily travel. Furthermore, results of this study indicate that using an integrated emission dispersion model for the assessment of individual exposure offers the opportunity for detailed, dynamic analysis of exposure to air pollution. The findings are useful for planning applications by using the model system to evaluate the effects of various transport policy scenarios on air pollution and exposure. In the last part of this research, a unique application was developed that supports decision makers by providing information about the current and future states of air pollution as a result of transit investments. For this purpose, the year 2008 was chosen as the base year and a future horizon year was chosen (2031). The integrated modelling system was used to evaluate the impact of an extensive regional transit improvement strategy revealing reductions in NO2 concentrations and exposures.
Near-road concentrations of nitrogen dioxide (NO2), a known marker of traffic-related air pollution, were simulated along a busy urban corridor in Montreal, Quebec using a combination of microscopic traffic simulation, instantaneous emission modeling, and air pollution dispersion. In order to calibrate and validate the model, measurements of NO2 were conducted mid-block along four segments of the corridor. The four segments were chosen to be consecutive and yet exhibiting variability in road configuration and built environment characteristics. Roadside NO2 measurements were also paired with on-site meteorological data collected using a portable meteorological station. In addition, traffic volumes, composition, and routing decisions were collected using video-cameras located at upstream and downstream intersections. Dispersion of simulated emissions was conducted for eight time slots and under a range of meteorological conditions using three different models with vastly different dispersion algorithms (OSPM, CALINE 4, and SIRANE). While the use of OSPM and SIRANE led to simulated NO2 concentrations closer to measured concentrations on road segments with buildings on both sides, CALINE 4 led to a better match with measured data when the built environment involves open terrain.
The objective of this thesis is to validate land-use regression models developed based on fixed and mobile measurements of ambient nitrogen dioxide (NO2) and ozone (O3) concentrations. For this purpose, a mobile monitoring campaign was conducted in the summer of 2015 in Montreal. The pollutant levels of 1,411 road segments were measured. Using data from repeated visits at each segment (N_vis), various land-use regression (LUR) models were developed based on segments with N_vis greater than or equal to 4, 8, 12, 16 and 20. Exposure surfaces for the island of Montreal based on these models were also developed.
Previously, during the summer of 2014, a monitoring campaign had taken place at 76 fixed locations spread around Montreal using the same sensors to measure the same two pollutants. LUR models as well as associated exposure surfaces were developed, and compared to the results from summer 2015.
We observed that the exposure surfaces resulting from both campaigns were highly dissimilar, and several possible explanations can be suggested. LUR models based on segments with a small number of repeated observations are associated with poor coefficients of determination (R²) and the exposure surfaces derived from them are poorly correlated with the summer 2014 exposure surface. On the other hand, restricting the LUR models to the road segments with N_vis greater than or equal to 16 leads to higher R² values at the expense of poor predictive capability outside of the sample mainly due to the fact that as N_vis increases, the variability in segment attributes within the sub-sample decreases as those segments become more concentrated in the downtown area.
This study highlights the sensitivity of LUR models based on mobile monitoring campaigns to the number of visits per segment and to the location of the segments and stresses the importance of careful design of outdoor data collection campaigns.
This research is motivated by the importance of reducing transit bus emissions in urban areas which can only be achieved after understanding the factors affecting transit bus emissions and exploring the effects of various emission reduction strategies. We started with understanding the emissions estimation methods currently embedded within emission inventory models followed by a validation of the most commonly used model in North America, the Motor Vehicle Emissions Simulator (MOVES), in a local context by collecting instantaneous bus speed and passenger ridership data across a variety of transit routes (downtown and suburban/highway) and bus types (standard, articulated, old, and new). We then tested the effects of incorporating local drive cycles into the MOVES model illustrating the gains in accuracy that are achieved by replacing default drive-cycles with local ones. We analyzed the spatial and temporal variably of transit bus emissions across the island of Montreal and investigated the isolated and combined effects of different factors affecting emissions (including the level of congestion, roadway grade, passenger ridership, and traffic variability). We also investigated the reduction potential of different fuels including ultra-low sulfur diesel, compressed natural gas; and of transit service operational improvements including transit signal priority, queue jumper lane, and relocation of bus stops considering a wide combination of congestion, roadway grade, and passenger ridership. Finally, a corridor study was conducted to capture the changes in emissions as a result of the implementation of different transit service improvement strategies including smart card, express bus service, and reserved bus lane. In order to assess whether the emission reductions observed for transit buses could translate to passenger cars, we estimated passenger vehicle emissions in a large Montreal neighbourhood and investigated the potential of different emission reduction strategies. This thesis addresses critical gaps in the current knowledge of transit bus emissions, providing useful information to transit planners when implementing emission reduction strategies or modifying existing transit facilities.
This thesis aims at developing air pollution exposure surfaces for nitrogen dioxide (NO2) and ozone (O3) in Montreal, Canada, using land use regression techniques, and thus capturing the effects of the built environment, traffic, and meteorology on the spatial variability of these two pollutants. Another objective of this work is to assess the potential of new handheld air quality sensors at capturing near-road air quality. To reach these objectives, we ran a data collection campaign on the island of Montreal, spanning 3 seasons (spring, summer and fall) in 2014. In total, 76 sites were identified and which represent the range of land-use and built environment characteristics in Montreal. Each site was visited at least 6 times throughout the campaign whereby measurements occurred for 30 minutes (per visit) with two O3 and two NO2 monitors. We also collected variables related to traffic and street characteristics on-site to assess their relationship with air pollution, and gathered a set of land-use and built environment characteristics at several buffer sizes around the sites (50, 100, 200, 300, 500, 750, 1000 m) using geographical information systems (GIS). The land use regression models we developed achieved R2 values of 0.86 for NO2 and 0.92 for O3, when corrected for regional meteorology. Based on the coefficients of the NO2 and O3 models, we developed an exposure surface for each pollutant by applying the model in areas where air pollution data were not available. Our NO2 surface is strongly correlated with older surfaces previously developed for Montreal, indicating that the spatial variability of air pollution has remained stable. Our O3 model is to our knowledge the first of its kind and for this reason cannot be compared with previous results. The relationship between NO2 and O3 in our models, confirms our theoretical understanding of the behaviour of these pollutants. The exposure we generated in this study can be used for epidemiological studies in evaluating the associations between traffic related air pollution and health.
The objective of this thesis is to identify factors related to meteorology and the built environment and its influence on near-roadway concentrations of ultrafine particles (UFP) in the context of restaurants and bars located within public open air spaces. The question that must be posed is how are variations in weather, traffic, on-site building characteristics impacting levels of UFP concentration, which in turn may produce adverse health effects for restaurant patrons as well as individuals working within hospitality industries.
To facilitate this study, a UFP monitoring campaign was organized and conducted during the months of May to July to measure at eight selected sites situated in Montreal, Canada. The eight study sites, chosen to reflect varying land use compositions and building characteristics, were each visited four times during lunch and dinner hours, where a site would be visited a total of two times (once per time period) during the weekday, and two times (once per time period) during the weekend. This campaign used meteorological information collected at the Montreal-Pierre Elliott Trudeau International Airport and at an automated weather station reporting from downtown Montreal. Traffic-related variables were derived from manual vehicle counts carried out at each study location, and the analysis of land use and other built characteristics relied on geographic information systems (GIS) data extracted from Transportation Research at McGill (TRAM), a transportation research group based at McGill University.
The investigation following the conclusion of the data collection campaign began by developing linear mixed effect models centred on variables relating to meteorology, traffic, site characteristics, and temporal factors. This data enables the validation of trends already defined in previous academic literature as well as to identify key findings that are contrary to intuitive hypotheses in the context of this study. Of note, meteorological effects such as temperature, wind speed, and wind direction relative to the orientation of the street are proven to possess inverse relationships with UFP. Homogeneity of land use is also an influential parameter and of significance with respect to this study of mixed use microenvironments. Furthermore, traffic-based predictors, most notably that of total diesel vehicles, contributed marginally to their concentrations.
The conclusions reached from this study, along with what is already known about ultrafine particles, allows for dialogue pertaining to optimizing the effectiveness of mixed use neighbourhoods from a health perspective in the context of open air patios. Provisional recommendations to improve the decision-making processes involved with planning and engineering such neighbourhoods are discussed and is critical for understanding the health and well-being of urban residents.
This study aimed at capturing the determinants of near-road concentrations of ultrafine particles (UFP) using linear mixed-effects models, investigating the effects of meteorology, built environment, and traffic. In addition, the differences in the levels of UFP between both sides of the road were investigated. To reach these objectives, field measurements were conducted on 16 weekdays in the months of March and April 2015, along Papineau Avenue, a high-volume street in Montreal, Canada. Four sites were identified varying in land use, building height, and road characteristics. Air quality measurements were conducted at each location (on both sides of the road) for two consecutive hours, at four different times during the day and repeated four times, leading to a total of 16 visits per location. Traffic volume and composition was also recorded. On-site meteorological variables including wind speed, wind direction, temperature and relative humidity were collected using a portable weather station. Linear mixed-effects models with random intercept were developed for both dependent variables: the natural logarithm of the mean UFP concentration and the difference in UFP concentrations between two sides of the road. Lower temperatures and wind speeds were associated with increased UFP concentrations. Winds orthogonal to the road tended to increase UFP concentrations as well as the differences between both sides of the road. Finally, built environment variables such as the presence of open areas and buildings on both sides of the road, had a positive influence on the difference between UFP on the two sides.
This thesis seeks to understand how the built environment and surrounding land use affect variations in near-roadway air pollution. In particular, two air pollutants are investigated: ultrafine particles (UFP) and black carbon (BC). Specifically, the study explores this question in the context of urban cycling. That is, how do factors such as traffic, buildings, and cycling infrastructure affect the concentration of air pollution to which cyclists are exposed?These answers are sought by way of a large-scale environmental monitoring campaign on the Island of Montreal during the summer of 2012. The campaign is comprised of two components: a mobile measurement portion whereby bicycles equipped with air pollution monitoring equipment cycle across roughly 500 km of unique roadway collecting UFP and BC concentrations, paired with global positioning system (GPS) data which allowed air pollution levels to be associated with the street on which they were collected—and a fixed site monitoring portion whereby research assistants measured pollution and counted traffic volumes and composition for set intervals of time at 73 locations. The overarching objective was to explain the variations in air pollution concentration by meteorological and built environment data, using land-use regression (LUR) analysis techniques. The mobile analysis relies primarily on geographic information systems (GIS) based land-use data while the fixed site analysis relies primarily on field measurements.Several investigations follow from this general data collection campaign. From the fixed site data a LUR model is developed based on meteorological factors, vehicular volumes and compositions, and built environment characteristics of the roadway corridor. These data also form the basis of a secondary investigation which explains the differences in UFP levels on opposite sides of the same street using wind, urban canyon, and traffic characteristics. Notable findings include support for some meteorological and urban canyon effects on air pollution, the relevance of both vehicular volumes as well as the truck component thereof.Two investigations arise from the mobile data collection campaign. The first attempts to explain the variations in air pollution using meteorological, land-use, and roadway characteristics, including results from a mesoscopic traffic simulation. The second seeks to understand how the nature of cycling infrastructure and cycling network design affect cyclists’ exposure to pollution. In addition to the effects captured in the fixed site analysis, relevant observations include the strong effects of nearby highways, especially for BC, and nearby restaurants, especially for UFP. The latter investigation from the mobile campaign shows that cycling facilities along major roads tend to have higher levels of pollution, however separated cycling infrastructure did reduce exposure to BC, perhaps owing in part from their greater distance from the street centerline. However the strongest reductions in air pollution were observed on multi-use trails, which typically run through parks and are located at substantial distances from the street.Together, these investigations use a novel methodological framework to unravel the interactions between cycling, the built environment, land use, traffic, and air pollution.
Road transport has a tremendous impact on local urban regions as well as global planetary health. This impact is especially great given the large quantities of greenhouse gases and local air pollutants released across the world, quantities that continue to increase. For metropolitan regions, reductions in traffic-related air pollution are paramount. Which baseline is used and which strategies should be implemented are both vital questions in this regard. Integrated transport and emissions models are important tools that aid metropolitan planners in answering those questions. A regional traffic assignment model has been connected to a detailed emission processor for the Montreal metropolitan region. The road transport model contains details on all private driving trips across a standard 24-hr workday, including congested link speeds and stochastic path distributions. Meanwhile, the emissions processor incorporates local vehicle registry data and Montreal-specific ambient conditions in the estimation of both running and start emissions. Outputs include hourly link-level and trip-level emissions for greenhouse gases, hydrocarbons, and nitrogen oxides. Three research studies were then explored that were anchored by the integrated transport and emissions model. The first involved testing model sensitivity to variations in input data and randomness. The second study was aimed at understanding the land-use and socioeconomic determinants of traffic-related air pollution generation and exposure. The third study encompassed an equity analysis of social disadvantage, traffic-related air pollution generation and exposure. Major findings include evidence that: start emissions and accurate vehicle registry data have the biggest impact on accurate regional emission inventories; neighbourhoods closer to downtown tend to be low emitters while having high exposures to traffic-related air pollution, while the opposite is true for neighbourhoods in the suburbs and periphery of the region; and marginalized neighbourhoods with high social disadvantage tend to have the highest exposure levels in the region, while at the same time generating some of the lowest quantities of traffic-related air pollution. These findings support the claim that traffic is creating environmental justice issues at the metropolitan level.
This thesis focuses on the development of a microscopic traffic simulation, emission and dispersion modeling system which aims at quantifying the effects of different types of traffic calming measures on vehicle emissions both at a link-level and at a network-level and on air quality at a corridor level using a scenario analysis. The study area is set in Montréal, Canada where a traffic simulation model for a dense urban neighborhood is extended with capabilities for microscopic emission estimation and dispersion modeling. The results indicate that on average, isolated calming measures increase carbon dioxide (CO2), carbon monoxide (CO) and nitrogen oxide (NOx) emissions by 1.50%, 0.33% and 1.45%, respectively across the entire network. Area-wide schemes result in a percentage increase of 3.84% for CO2, 1.22% for CO, and 2.18% for NOx. Along specific corridors where traffic calming measures were simulated, increases in CO2 emissions of up to 83% are observed. These increases are mainly associated with a change in vehicle drive-cycles through increased accelerations and decelerations. The results for air quality modeling suggest on average NO2 levels increase between 0.1% and 10% with respect to the base case. A high positive correlation of 0.7 between segment emissions of NOx and concentrations of NO2 is observed. Also, the effects of wind speed and direction are investigated in this thesis. The results show that higher wind speeds decrease NO2 concentrations on both sides of the roadway while winds orthogonal to the road increase the difference between concentrations on the leeward and windward sides with the leeward side experiencing higher levels. The effect of different measures on traffic volumes is also investigated and moderate decreases in areas that have undergone traffic calming are observed. Finally, the results show that speed bumps result in higher emission levels and poorer near-roadway air quality than speed humps.